
An official website of the United States government
The .gov means it’s official. Federal government websites often end in .gov or .mil. Before sharing sensitive information, make sure you’re on a federal government site.
The site is secure. The https:// ensures that you are connecting to the official website and that any information you provide is encrypted and transmitted securely.
- Publications
- Account settings
Preview improvements coming to the PMC website in October 2024. Learn More or Try it out now .
- Advanced Search
- Journal List
- CBE Life Sci Educ
- v.17(1); Spring 2018

Understanding the Complex Relationship between Critical Thinking and Science Reasoning among Undergraduate Thesis Writers
Jason e. dowd.
† Department of Biology, Duke University, Durham, NC 27708
Robert J. Thompson, Jr.
‡ Department of Psychology and Neuroscience, Duke University, Durham, NC 27708
Leslie A. Schiff
§ Department of Microbiology and Immunology, University of Minnesota, Minneapolis, MN 55455
Julie A. Reynolds
Associated data.
This study empirically examines the relationship between students’ critical-thinking skills and scientific reasoning as reflected in undergraduate thesis writing in biology. Writing offers a unique window into studying this relationship, and the findings raise potential implications for instruction.
Developing critical-thinking and scientific reasoning skills are core learning objectives of science education, but little empirical evidence exists regarding the interrelationships between these constructs. Writing effectively fosters students’ development of these constructs, and it offers a unique window into studying how they relate. In this study of undergraduate thesis writing in biology at two universities, we examine how scientific reasoning exhibited in writing (assessed using the Biology Thesis Assessment Protocol) relates to general and specific critical-thinking skills (assessed using the California Critical Thinking Skills Test), and we consider implications for instruction. We find that scientific reasoning in writing is strongly related to inference , while other aspects of science reasoning that emerge in writing (epistemological considerations, writing conventions, etc.) are not significantly related to critical-thinking skills. Science reasoning in writing is not merely a proxy for critical thinking. In linking features of students’ writing to their critical-thinking skills, this study 1) provides a bridge to prior work suggesting that engagement in science writing enhances critical thinking and 2) serves as a foundational step for subsequently determining whether instruction focused explicitly on developing critical-thinking skills (particularly inference ) can actually improve students’ scientific reasoning in their writing.
INTRODUCTION
Critical-thinking and scientific reasoning skills are core learning objectives of science education for all students, regardless of whether or not they intend to pursue a career in science or engineering. Consistent with the view of learning as construction of understanding and meaning ( National Research Council, 2000 ), the pedagogical practice of writing has been found to be effective not only in fostering the development of students’ conceptual and procedural knowledge ( Gerdeman et al. , 2007 ) and communication skills ( Clase et al. , 2010 ), but also scientific reasoning ( Reynolds et al. , 2012 ) and critical-thinking skills ( Quitadamo and Kurtz, 2007 ).
Critical thinking and scientific reasoning are similar but different constructs that include various types of higher-order cognitive processes, metacognitive strategies, and dispositions involved in making meaning of information. Critical thinking is generally understood as the broader construct ( Holyoak and Morrison, 2005 ), comprising an array of cognitive processes and dispostions that are drawn upon differentially in everyday life and across domains of inquiry such as the natural sciences, social sciences, and humanities. Scientific reasoning, then, may be interpreted as the subset of critical-thinking skills (cognitive and metacognitive processes and dispositions) that 1) are involved in making meaning of information in scientific domains and 2) support the epistemological commitment to scientific methodology and paradigm(s).
Although there has been an enduring focus in higher education on promoting critical thinking and reasoning as general or “transferable” skills, research evidence provides increasing support for the view that reasoning and critical thinking are also situational or domain specific ( Beyer et al. , 2013 ). Some researchers, such as Lawson (2010) , present frameworks in which science reasoning is characterized explicitly in terms of critical-thinking skills. There are, however, limited coherent frameworks and empirical evidence regarding either the general or domain-specific interrelationships of scientific reasoning, as it is most broadly defined, and critical-thinking skills.
The Vision and Change in Undergraduate Biology Education Initiative provides a framework for thinking about these constructs and their interrelationship in the context of the core competencies and disciplinary practice they describe ( American Association for the Advancement of Science, 2011 ). These learning objectives aim for undergraduates to “understand the process of science, the interdisciplinary nature of the new biology and how science is closely integrated within society; be competent in communication and collaboration; have quantitative competency and a basic ability to interpret data; and have some experience with modeling, simulation and computational and systems level approaches as well as with using large databases” ( Woodin et al. , 2010 , pp. 71–72). This framework makes clear that science reasoning and critical-thinking skills play key roles in major learning outcomes; for example, “understanding the process of science” requires students to engage in (and be metacognitive about) scientific reasoning, and having the “ability to interpret data” requires critical-thinking skills. To help students better achieve these core competencies, we must better understand the interrelationships of their composite parts. Thus, the next step is to determine which specific critical-thinking skills are drawn upon when students engage in science reasoning in general and with regard to the particular scientific domain being studied. Such a determination could be applied to improve science education for both majors and nonmajors through pedagogical approaches that foster critical-thinking skills that are most relevant to science reasoning.
Writing affords one of the most effective means for making thinking visible ( Reynolds et al. , 2012 ) and learning how to “think like” and “write like” disciplinary experts ( Meizlish et al. , 2013 ). As a result, student writing affords the opportunities to both foster and examine the interrelationship of scientific reasoning and critical-thinking skills within and across disciplinary contexts. The purpose of this study was to better understand the relationship between students’ critical-thinking skills and scientific reasoning skills as reflected in the genre of undergraduate thesis writing in biology departments at two research universities, the University of Minnesota and Duke University.
In the following subsections, we discuss in greater detail the constructs of scientific reasoning and critical thinking, as well as the assessment of scientific reasoning in students’ thesis writing. In subsequent sections, we discuss our study design, findings, and the implications for enhancing educational practices.
Critical Thinking
The advances in cognitive science in the 21st century have increased our understanding of the mental processes involved in thinking and reasoning, as well as memory, learning, and problem solving. Critical thinking is understood to include both a cognitive dimension and a disposition dimension (e.g., reflective thinking) and is defined as “purposeful, self-regulatory judgment which results in interpretation, analysis, evaluation, and inference, as well as explanation of the evidential, conceptual, methodological, criteriological, or contextual considerations upon which that judgment is based” ( Facione, 1990, p. 3 ). Although various other definitions of critical thinking have been proposed, researchers have generally coalesced on this consensus: expert view ( Blattner and Frazier, 2002 ; Condon and Kelly-Riley, 2004 ; Bissell and Lemons, 2006 ; Quitadamo and Kurtz, 2007 ) and the corresponding measures of critical-thinking skills ( August, 2016 ; Stephenson and Sadler-McKnight, 2016 ).
Both the cognitive skills and dispositional components of critical thinking have been recognized as important to science education ( Quitadamo and Kurtz, 2007 ). Empirical research demonstrates that specific pedagogical practices in science courses are effective in fostering students’ critical-thinking skills. Quitadamo and Kurtz (2007) found that students who engaged in a laboratory writing component in the context of a general education biology course significantly improved their overall critical-thinking skills (and their analytical and inference skills, in particular), whereas students engaged in a traditional quiz-based laboratory did not improve their critical-thinking skills. In related work, Quitadamo et al. (2008) found that a community-based inquiry experience, involving inquiry, writing, research, and analysis, was associated with improved critical thinking in a biology course for nonmajors, compared with traditionally taught sections. In both studies, students who exhibited stronger presemester critical-thinking skills exhibited stronger gains, suggesting that “students who have not been explicitly taught how to think critically may not reach the same potential as peers who have been taught these skills” ( Quitadamo and Kurtz, 2007 , p. 151).
Recently, Stephenson and Sadler-McKnight (2016) found that first-year general chemistry students who engaged in a science writing heuristic laboratory, which is an inquiry-based, writing-to-learn approach to instruction ( Hand and Keys, 1999 ), had significantly greater gains in total critical-thinking scores than students who received traditional laboratory instruction. Each of the four components—inquiry, writing, collaboration, and reflection—have been linked to critical thinking ( Stephenson and Sadler-McKnight, 2016 ). Like the other studies, this work highlights the value of targeting critical-thinking skills and the effectiveness of an inquiry-based, writing-to-learn approach to enhance critical thinking. Across studies, authors advocate adopting critical thinking as the course framework ( Pukkila, 2004 ) and developing explicit examples of how critical thinking relates to the scientific method ( Miri et al. , 2007 ).
In these examples, the important connection between writing and critical thinking is highlighted by the fact that each intervention involves the incorporation of writing into science, technology, engineering, and mathematics education (either alone or in combination with other pedagogical practices). However, critical-thinking skills are not always the primary learning outcome; in some contexts, scientific reasoning is the primary outcome that is assessed.
Scientific Reasoning
Scientific reasoning is a complex process that is broadly defined as “the skills involved in inquiry, experimentation, evidence evaluation, and inference that are done in the service of conceptual change or scientific understanding” ( Zimmerman, 2007 , p. 172). Scientific reasoning is understood to include both conceptual knowledge and the cognitive processes involved with generation of hypotheses (i.e., inductive processes involved in the generation of hypotheses and the deductive processes used in the testing of hypotheses), experimentation strategies, and evidence evaluation strategies. These dimensions are interrelated, in that “experimentation and inference strategies are selected based on prior conceptual knowledge of the domain” ( Zimmerman, 2000 , p. 139). Furthermore, conceptual and procedural knowledge and cognitive process dimensions can be general and domain specific (or discipline specific).
With regard to conceptual knowledge, attention has been focused on the acquisition of core methodological concepts fundamental to scientists’ causal reasoning and metacognitive distancing (or decontextualized thinking), which is the ability to reason independently of prior knowledge or beliefs ( Greenhoot et al. , 2004 ). The latter involves what Kuhn and Dean (2004) refer to as the coordination of theory and evidence, which requires that one question existing theories (i.e., prior knowledge and beliefs), seek contradictory evidence, eliminate alternative explanations, and revise one’s prior beliefs in the face of contradictory evidence. Kuhn and colleagues (2008) further elaborate that scientific thinking requires “a mature understanding of the epistemological foundations of science, recognizing scientific knowledge as constructed by humans rather than simply discovered in the world,” and “the ability to engage in skilled argumentation in the scientific domain, with an appreciation of argumentation as entailing the coordination of theory and evidence” ( Kuhn et al. , 2008 , p. 435). “This approach to scientific reasoning not only highlights the skills of generating and evaluating evidence-based inferences, but also encompasses epistemological appreciation of the functions of evidence and theory” ( Ding et al. , 2016 , p. 616). Evaluating evidence-based inferences involves epistemic cognition, which Moshman (2015) defines as the subset of metacognition that is concerned with justification, truth, and associated forms of reasoning. Epistemic cognition is both general and domain specific (or discipline specific; Moshman, 2015 ).
There is empirical support for the contributions of both prior knowledge and an understanding of the epistemological foundations of science to scientific reasoning. In a study of undergraduate science students, advanced scientific reasoning was most often accompanied by accurate prior knowledge as well as sophisticated epistemological commitments; additionally, for students who had comparable levels of prior knowledge, skillful reasoning was associated with a strong epistemological commitment to the consistency of theory with evidence ( Zeineddin and Abd-El-Khalick, 2010 ). These findings highlight the importance of the need for instructional activities that intentionally help learners develop sophisticated epistemological commitments focused on the nature of knowledge and the role of evidence in supporting knowledge claims ( Zeineddin and Abd-El-Khalick, 2010 ).
Scientific Reasoning in Students’ Thesis Writing
Pedagogical approaches that incorporate writing have also focused on enhancing scientific reasoning. Many rubrics have been developed to assess aspects of scientific reasoning in written artifacts. For example, Timmerman and colleagues (2011) , in the course of describing their own rubric for assessing scientific reasoning, highlight several examples of scientific reasoning assessment criteria ( Haaga, 1993 ; Tariq et al. , 1998 ; Topping et al. , 2000 ; Kelly and Takao, 2002 ; Halonen et al. , 2003 ; Willison and O’Regan, 2007 ).
At both the University of Minnesota and Duke University, we have focused on the genre of the undergraduate honors thesis as the rhetorical context in which to study and improve students’ scientific reasoning and writing. We view the process of writing an undergraduate honors thesis as a form of professional development in the sciences (i.e., a way of engaging students in the practices of a community of discourse). We have found that structured courses designed to scaffold the thesis-writing process and promote metacognition can improve writing and reasoning skills in biology, chemistry, and economics ( Reynolds and Thompson, 2011 ; Dowd et al. , 2015a , b ). In the context of this prior work, we have defined scientific reasoning in writing as the emergent, underlying construct measured across distinct aspects of students’ written discussion of independent research in their undergraduate theses.
The Biology Thesis Assessment Protocol (BioTAP) was developed at Duke University as a tool for systematically guiding students and faculty through a “draft–feedback–revision” writing process, modeled after professional scientific peer-review processes ( Reynolds et al. , 2009 ). BioTAP includes activities and worksheets that allow students to engage in critical peer review and provides detailed descriptions, presented as rubrics, of the questions (i.e., dimensions, shown in Table 1 ) upon which such review should focus. Nine rubric dimensions focus on communication to the broader scientific community, and four rubric dimensions focus on the accuracy and appropriateness of the research. These rubric dimensions provide criteria by which the thesis is assessed, and therefore allow BioTAP to be used as an assessment tool as well as a teaching resource ( Reynolds et al. , 2009 ). Full details are available at www.science-writing.org/biotap.html .
Theses assessment protocol dimensions
In previous work, we have used BioTAP to quantitatively assess students’ undergraduate honors theses and explore the relationship between thesis-writing courses (or specific interventions within the courses) and the strength of students’ science reasoning in writing across different science disciplines: biology ( Reynolds and Thompson, 2011 ); chemistry ( Dowd et al. , 2015b ); and economics ( Dowd et al. , 2015a ). We have focused exclusively on the nine dimensions related to reasoning and writing (questions 1–9), as the other four dimensions (questions 10–13) require topic-specific expertise and are intended to be used by the student’s thesis supervisor.
Beyond considering individual dimensions, we have investigated whether meaningful constructs underlie students’ thesis scores. We conducted exploratory factor analysis of students’ theses in biology, economics, and chemistry and found one dominant underlying factor in each discipline; we termed the factor “scientific reasoning in writing” ( Dowd et al. , 2015a , b , 2016 ). That is, each of the nine dimensions could be understood as reflecting, in different ways and to different degrees, the construct of scientific reasoning in writing. The findings indicated evidence of both general and discipline-specific components to scientific reasoning in writing that relate to epistemic beliefs and paradigms, in keeping with broader ideas about science reasoning discussed earlier. Specifically, scientific reasoning in writing is more strongly associated with formulating a compelling argument for the significance of the research in the context of current literature in biology, making meaning regarding the implications of the findings in chemistry, and providing an organizational framework for interpreting the thesis in economics. We suggested that instruction, whether occurring in writing studios or in writing courses to facilitate thesis preparation, should attend to both components.
Research Question and Study Design
The genre of thesis writing combines the pedagogies of writing and inquiry found to foster scientific reasoning ( Reynolds et al. , 2012 ) and critical thinking ( Quitadamo and Kurtz, 2007 ; Quitadamo et al. , 2008 ; Stephenson and Sadler-McKnight, 2016 ). However, there is no empirical evidence regarding the general or domain-specific interrelationships of scientific reasoning and critical-thinking skills, particularly in the rhetorical context of the undergraduate thesis. The BioTAP studies discussed earlier indicate that the rubric-based assessment produces evidence of scientific reasoning in the undergraduate thesis, but it was not designed to foster or measure critical thinking. The current study was undertaken to address the research question: How are students’ critical-thinking skills related to scientific reasoning as reflected in the genre of undergraduate thesis writing in biology? Determining these interrelationships could guide efforts to enhance students’ scientific reasoning and writing skills through focusing instruction on specific critical-thinking skills as well as disciplinary conventions.
To address this research question, we focused on undergraduate thesis writers in biology courses at two institutions, Duke University and the University of Minnesota, and examined the extent to which students’ scientific reasoning in writing, assessed in the undergraduate thesis using BioTAP, corresponds to students’ critical-thinking skills, assessed using the California Critical Thinking Skills Test (CCTST; August, 2016 ).
Study Sample
The study sample was composed of students enrolled in courses designed to scaffold the thesis-writing process in the Department of Biology at Duke University and the College of Biological Sciences at the University of Minnesota. Both courses complement students’ individual work with research advisors. The course is required for thesis writers at the University of Minnesota and optional for writers at Duke University. Not all students are required to complete a thesis, though it is required for students to graduate with honors; at the University of Minnesota, such students are enrolled in an honors program within the college. In total, 28 students were enrolled in the course at Duke University and 44 students were enrolled in the course at the University of Minnesota. Of those students, two students did not consent to participate in the study; additionally, five students did not validly complete the CCTST (i.e., attempted fewer than 60% of items or completed the test in less than 15 minutes). Thus, our overall rate of valid participation is 90%, with 27 students from Duke University and 38 students from the University of Minnesota. We found no statistically significant differences in thesis assessment between students with valid CCTST scores and invalid CCTST scores. Therefore, we focus on the 65 students who consented to participate and for whom we have complete and valid data in most of this study. Additionally, in asking students for their consent to participate, we allowed them to choose whether to provide or decline access to academic and demographic background data. Of the 65 students who consented to participate, 52 students granted access to such data. Therefore, for additional analyses involving academic and background data, we focus on the 52 students who consented. We note that the 13 students who participated but declined to share additional data performed slightly lower on the CCTST than the 52 others (perhaps suggesting that they differ by other measures, but we cannot determine this with certainty). Among the 52 students, 60% identified as female and 10% identified as being from underrepresented ethnicities.
In both courses, students completed the CCTST online, either in class or on their own, late in the Spring 2016 semester. This is the same assessment that was used in prior studies of critical thinking ( Quitadamo and Kurtz, 2007 ; Quitadamo et al. , 2008 ; Stephenson and Sadler-McKnight, 2016 ). It is “an objective measure of the core reasoning skills needed for reflective decision making concerning what to believe or what to do” ( Insight Assessment, 2016a ). In the test, students are asked to read and consider information as they answer multiple-choice questions. The questions are intended to be appropriate for all users, so there is no expectation of prior disciplinary knowledge in biology (or any other subject). Although actual test items are protected, sample items are available on the Insight Assessment website ( Insight Assessment, 2016b ). We have included one sample item in the Supplemental Material.
The CCTST is based on a consensus definition of critical thinking, measures cognitive and metacognitive skills associated with critical thinking, and has been evaluated for validity and reliability at the college level ( August, 2016 ; Stephenson and Sadler-McKnight, 2016 ). In addition to providing overall critical-thinking score, the CCTST assesses seven dimensions of critical thinking: analysis, interpretation, inference, evaluation, explanation, induction, and deduction. Scores on each dimension are calculated based on students’ performance on items related to that dimension. Analysis focuses on identifying assumptions, reasons, and claims and examining how they interact to form arguments. Interpretation, related to analysis, focuses on determining the precise meaning and significance of information. Inference focuses on drawing conclusions from reasons and evidence. Evaluation focuses on assessing the credibility of sources of information and claims they make. Explanation, related to evaluation, focuses on describing the evidence, assumptions, or rationale for beliefs and conclusions. Induction focuses on drawing inferences about what is probably true based on evidence. Deduction focuses on drawing conclusions about what must be true when the context completely determines the outcome. These are not independent dimensions; the fact that they are related supports their collective interpretation as critical thinking. Together, the CCTST dimensions provide a basis for evaluating students’ overall strength in using reasoning to form reflective judgments about what to believe or what to do ( August, 2016 ). Each of the seven dimensions and the overall CCTST score are measured on a scale of 0–100, where higher scores indicate superior performance. Scores correspond to superior (86–100), strong (79–85), moderate (70–78), weak (63–69), or not manifested (62 and below) skills.
Scientific Reasoning in Writing
At the end of the semester, students’ final, submitted undergraduate theses were assessed using BioTAP, which consists of nine rubric dimensions that focus on communication to the broader scientific community and four additional dimensions that focus on the exhibition of topic-specific expertise ( Reynolds et al. , 2009 ). These dimensions, framed as questions, are displayed in Table 1 .
Student theses were assessed on questions 1–9 of BioTAP using the same procedures described in previous studies ( Reynolds and Thompson, 2011 ; Dowd et al. , 2015a , b ). In this study, six raters were trained in the valid, reliable use of BioTAP rubrics. Each dimension was rated on a five-point scale: 1 indicates the dimension is missing, incomplete, or below acceptable standards; 3 indicates that the dimension is adequate but not exhibiting mastery; and 5 indicates that the dimension is excellent and exhibits mastery (intermediate ratings of 2 and 4 are appropriate when different parts of the thesis make a single category challenging). After training, two raters independently assessed each thesis and then discussed their independent ratings with one another to form a consensus rating. The consensus score is not an average score, but rather an agreed-upon, discussion-based score. On a five-point scale, raters independently assessed dimensions to be within 1 point of each other 82.4% of the time before discussion and formed consensus ratings 100% of the time after discussion.
In this study, we consider both categorical (mastery/nonmastery, where a score of 5 corresponds to mastery) and numerical treatments of individual BioTAP scores to better relate the manifestation of critical thinking in BioTAP assessment to all of the prior studies. For comprehensive/cumulative measures of BioTAP, we focus on the partial sum of questions 1–5, as these questions relate to higher-order scientific reasoning (whereas questions 6–9 relate to mid- and lower-order writing mechanics [ Reynolds et al. , 2009 ]), and the factor scores (i.e., numerical representations of the extent to which each student exhibits the underlying factor), which are calculated from the factor loadings published by Dowd et al. (2016) . We do not focus on questions 6–9 individually in statistical analyses, because we do not expect critical-thinking skills to relate to mid- and lower-order writing skills.
The final, submitted thesis reflects the student’s writing, the student’s scientific reasoning, the quality of feedback provided to the student by peers and mentors, and the student’s ability to incorporate that feedback into his or her work. Therefore, our assessment is not the same as an assessment of unpolished, unrevised samples of students’ written work. While one might imagine that such an unpolished sample may be more strongly correlated with critical-thinking skills measured by the CCTST, we argue that the complete, submitted thesis, assessed using BioTAP, is ultimately a more appropriate reflection of how students exhibit science reasoning in the scientific community.
Statistical Analyses
We took several steps to analyze the collected data. First, to provide context for subsequent interpretations, we generated descriptive statistics for the CCTST scores of the participants based on the norms for undergraduate CCTST test takers. To determine the strength of relationships among CCTST dimensions (including overall score) and the BioTAP dimensions, partial-sum score (questions 1–5), and factor score, we calculated Pearson’s correlations for each pair of measures. To examine whether falling on one side of the nonmastery/mastery threshold (as opposed to a linear scale of performance) was related to critical thinking, we grouped BioTAP dimensions into categories (mastery/nonmastery) and conducted Student’s t tests to compare the means scores of the two groups on each of the seven dimensions and overall score of the CCTST. Finally, for the strongest relationship that emerged, we included additional academic and background variables as covariates in multiple linear-regression analysis to explore questions about how much observed relationships between critical-thinking skills and science reasoning in writing might be explained by variation in these other factors.
Although BioTAP scores represent discreet, ordinal bins, the five-point scale is intended to capture an underlying continuous construct (from inadequate to exhibiting mastery). It has been argued that five categories is an appropriate cutoff for treating ordinal variables as pseudo-continuous ( Rhemtulla et al. , 2012 )—and therefore using continuous-variable statistical methods (e.g., Pearson’s correlations)—as long as the underlying assumption that ordinal scores are linearly distributed is valid. Although we have no way to statistically test this assumption, we interpret adequate scores to be approximately halfway between inadequate and mastery scores, resulting in a linear scale. In part because this assumption is subject to disagreement, we also consider and interpret a categorical (mastery/nonmastery) treatment of BioTAP variables.
We corrected for multiple comparisons using the Holm-Bonferroni method ( Holm, 1979 ). At the most general level, where we consider the single, comprehensive measures for BioTAP (partial-sum and factor score) and the CCTST (overall score), there is no need to correct for multiple comparisons, because the multiple, individual dimensions are collapsed into single dimensions. When we considered individual CCTST dimensions in relation to comprehensive measures for BioTAP, we accounted for seven comparisons; similarly, when we considered individual dimensions of BioTAP in relation to overall CCTST score, we accounted for five comparisons. When all seven CCTST and five BioTAP dimensions were examined individually and without prior knowledge, we accounted for 35 comparisons; such a rigorous threshold is likely to reject weak and moderate relationships, but it is appropriate if there are no specific pre-existing hypotheses. All p values are presented in tables for complete transparency, and we carefully consider the implications of our interpretation of these data in the Discussion section.
CCTST scores for students in this sample ranged from the 39th to 99th percentile of the general population of undergraduate CCTST test takers (mean percentile = 84.3, median = 85th percentile; Table 2 ); these percentiles reflect overall scores that range from moderate to superior. Scores on individual dimensions and overall scores were sufficiently normal and far enough from the ceiling of the scale to justify subsequent statistical analyses.
Descriptive statistics of CCTST dimensions a
Minimum | Mean | Median | Maximum | |
---|---|---|---|---|
Analysis | 70 | 88.6 | 90 | 100 |
Interpretation | 74 | 89.7 | 87 | 100 |
Inference | 78 | 87.9 | 89 | 100 |
Evaluation | 63 | 83.6 | 84 | 100 |
Explanation | 61 | 84.4 | 87 | 100 |
Induction | 74 | 87.4 | 87 | 97 |
Deduction | 71 | 86.4 | 87 | 97 |
Overall | 73 | 86 | 85 | 97 |
a Scores correspond to superior (86–100), strong (79–85), moderate (70–78), weak (63–69), or not manifested (62 and lower) skills.
The Pearson’s correlations between students’ cumulative scores on BioTAP (the factor score based on loadings published by Dowd et al. , 2016 , and the partial sum of scores on questions 1–5) and students’ overall scores on the CCTST are presented in Table 3 . We found that the partial-sum measure of BioTAP was significantly related to the overall measure of critical thinking ( r = 0.27, p = 0.03), while the BioTAP factor score was marginally related to overall CCTST ( r = 0.24, p = 0.05). When we looked at relationships between comprehensive BioTAP measures and scores for individual dimensions of the CCTST ( Table 3 ), we found significant positive correlations between the both BioTAP partial-sum and factor scores and CCTST inference ( r = 0.45, p < 0.001, and r = 0.41, p < 0.001, respectively). Although some other relationships have p values below 0.05 (e.g., the correlations between BioTAP partial-sum scores and CCTST induction and interpretation scores), they are not significant when we correct for multiple comparisons.
Correlations between dimensions of CCTST and dimensions of BioTAP a
a In each cell, the top number is the correlation, and the bottom, italicized number is the associated p value. Correlations that are statistically significant after correcting for multiple comparisons are shown in bold.
b This is the partial sum of BioTAP scores on questions 1–5.
c This is the factor score calculated from factor loadings published by Dowd et al. (2016) .
When we expanded comparisons to include all 35 potential correlations among individual BioTAP and CCTST dimensions—and, accordingly, corrected for 35 comparisons—we did not find any additional statistically significant relationships. The Pearson’s correlations between students’ scores on each dimension of BioTAP and students’ scores on each dimension of the CCTST range from −0.11 to 0.35 ( Table 3 ); although the relationship between discussion of implications (BioTAP question 5) and inference appears to be relatively large ( r = 0.35), it is not significant ( p = 0.005; the Holm-Bonferroni cutoff is 0.00143). We found no statistically significant relationships between BioTAP questions 6–9 and CCTST dimensions (unpublished data), regardless of whether we correct for multiple comparisons.
The results of Student’s t tests comparing scores on each dimension of the CCTST of students who exhibit mastery with those of students who do not exhibit mastery on each dimension of BioTAP are presented in Table 4 . Focusing first on the overall CCTST scores, we found that the difference between those who exhibit mastery and those who do not in discussing implications of results (BioTAP question 5) is statistically significant ( t = 2.73, p = 0.008, d = 0.71). When we expanded t tests to include all 35 comparisons—and, like above, corrected for 35 comparisons—we found a significant difference in inference scores between students who exhibit mastery on question 5 and students who do not ( t = 3.41, p = 0.0012, d = 0.88), as well as a marginally significant difference in these students’ induction scores ( t = 3.26, p = 0.0018, d = 0.84; the Holm-Bonferroni cutoff is p = 0.00147). Cohen’s d effect sizes, which reveal the strength of the differences for statistically significant relationships, range from 0.71 to 0.88.
The t statistics and effect sizes of differences in dimensions of CCTST across dimensions of BioTAP a
a In each cell, the top number is the t statistic for each comparison, and the middle, italicized number is the associated p value. The bottom number is the effect size. Correlations that are statistically significant after correcting for multiple comparisons are shown in bold.
Finally, we more closely examined the strongest relationship that we observed, which was between the CCTST dimension of inference and the BioTAP partial-sum composite score (shown in Table 3 ), using multiple regression analysis ( Table 5 ). Focusing on the 52 students for whom we have background information, we looked at the simple relationship between BioTAP and inference (model 1), a robust background model including multiple covariates that one might expect to explain some part of the variation in BioTAP (model 2), and a combined model including all variables (model 3). As model 3 shows, the covariates explain very little variation in BioTAP scores, and the relationship between inference and BioTAP persists even in the presence of all of the covariates.
Partial sum (questions 1–5) of BioTAP scores ( n = 52)
Variable | Model 1 | Model 2 | Model 3 |
---|---|---|---|
CCTST inference | 0.536*** | 0.491** | |
Grade point average | 0.176 | 0.092 | |
Independent study courses | −0.087 | 0.001 | |
Writing-intensive courses | 0.131 | 0.021 | |
Institution | 0.329 | 0.115 | |
Male | 0.085 | 0.041 | |
Underrepresented group | −0.114 | −0.060 | |
Adjusted | 0.273 | −0. 022 | 0.195 |
** p < 0.01.
*** p < 0.001.
The aim of this study was to examine the extent to which the various components of scientific reasoning—manifested in writing in the genre of undergraduate thesis and assessed using BioTAP—draw on general and specific critical-thinking skills (assessed using CCTST) and to consider the implications for educational practices. Although science reasoning involves critical-thinking skills, it also relates to conceptual knowledge and the epistemological foundations of science disciplines ( Kuhn et al. , 2008 ). Moreover, science reasoning in writing , captured in students’ undergraduate theses, reflects habits, conventions, and the incorporation of feedback that may alter evidence of individuals’ critical-thinking skills. Our findings, however, provide empirical evidence that cumulative measures of science reasoning in writing are nonetheless related to students’ overall critical-thinking skills ( Table 3 ). The particularly significant roles of inference skills ( Table 3 ) and the discussion of implications of results (BioTAP question 5; Table 4 ) provide a basis for more specific ideas about how these constructs relate to one another and what educational interventions may have the most success in fostering these skills.
Our results build on previous findings. The genre of thesis writing combines pedagogies of writing and inquiry found to foster scientific reasoning ( Reynolds et al. , 2012 ) and critical thinking ( Quitadamo and Kurtz, 2007 ; Quitadamo et al. , 2008 ; Stephenson and Sadler-McKnight, 2016 ). Quitadamo and Kurtz (2007) reported that students who engaged in a laboratory writing component in a general education biology course significantly improved their inference and analysis skills, and Quitadamo and colleagues (2008) found that participation in a community-based inquiry biology course (that included a writing component) was associated with significant gains in students’ inference and evaluation skills. The shared focus on inference is noteworthy, because these prior studies actually differ from the current study; the former considered critical-thinking skills as the primary learning outcome of writing-focused interventions, whereas the latter focused on emergent links between two learning outcomes (science reasoning in writing and critical thinking). In other words, inference skills are impacted by writing as well as manifested in writing.
Inference focuses on drawing conclusions from argument and evidence. According to the consensus definition of critical thinking, the specific skill of inference includes several processes: querying evidence, conjecturing alternatives, and drawing conclusions. All of these activities are central to the independent research at the core of writing an undergraduate thesis. Indeed, a critical part of what we call “science reasoning in writing” might be characterized as a measure of students’ ability to infer and make meaning of information and findings. Because the cumulative BioTAP measures distill underlying similarities and, to an extent, suppress unique aspects of individual dimensions, we argue that it is appropriate to relate inference to scientific reasoning in writing . Even when we control for other potentially relevant background characteristics, the relationship is strong ( Table 5 ).
In taking the complementary view and focusing on BioTAP, when we compared students who exhibit mastery with those who do not, we found that the specific dimension of “discussing the implications of results” (question 5) differentiates students’ performance on several critical-thinking skills. To achieve mastery on this dimension, students must make connections between their results and other published studies and discuss the future directions of the research; in short, they must demonstrate an understanding of the bigger picture. The specific relationship between question 5 and inference is the strongest observed among all individual comparisons. Altogether, perhaps more than any other BioTAP dimension, this aspect of students’ writing provides a clear view of the role of students’ critical-thinking skills (particularly inference and, marginally, induction) in science reasoning.
While inference and discussion of implications emerge as particularly strongly related dimensions in this work, we note that the strongest contribution to “science reasoning in writing in biology,” as determined through exploratory factor analysis, is “argument for the significance of research” (BioTAP question 2, not question 5; Dowd et al. , 2016 ). Question 2 is not clearly related to critical-thinking skills. These findings are not contradictory, but rather suggest that the epistemological and disciplinary-specific aspects of science reasoning that emerge in writing through BioTAP are not completely aligned with aspects related to critical thinking. In other words, science reasoning in writing is not simply a proxy for those critical-thinking skills that play a role in science reasoning.
In a similar vein, the content-related, epistemological aspects of science reasoning, as well as the conventions associated with writing the undergraduate thesis (including feedback from peers and revision), may explain the lack of significant relationships between some science reasoning dimensions and some critical-thinking skills that might otherwise seem counterintuitive (e.g., BioTAP question 2, which relates to making an argument, and the critical-thinking skill of argument). It is possible that an individual’s critical-thinking skills may explain some variation in a particular BioTAP dimension, but other aspects of science reasoning and practice exert much stronger influence. Although these relationships do not emerge in our analyses, the lack of significant correlation does not mean that there is definitively no correlation. Correcting for multiple comparisons suppresses type 1 error at the expense of exacerbating type 2 error, which, combined with the limited sample size, constrains statistical power and makes weak relationships more difficult to detect. Ultimately, though, the relationships that do emerge highlight places where individuals’ distinct critical-thinking skills emerge most coherently in thesis assessment, which is why we are particularly interested in unpacking those relationships.
We recognize that, because only honors students submit theses at these institutions, this study sample is composed of a selective subset of the larger population of biology majors. Although this is an inherent limitation of focusing on thesis writing, links between our findings and results of other studies (with different populations) suggest that observed relationships may occur more broadly. The goal of improved science reasoning and critical thinking is shared among all biology majors, particularly those engaged in capstone research experiences. So while the implications of this work most directly apply to honors thesis writers, we provisionally suggest that all students could benefit from further study of them.
There are several important implications of this study for science education practices. Students’ inference skills relate to the understanding and effective application of scientific content. The fact that we find no statistically significant relationships between BioTAP questions 6–9 and CCTST dimensions suggests that such mid- to lower-order elements of BioTAP ( Reynolds et al. , 2009 ), which tend to be more structural in nature, do not focus on aspects of the finished thesis that draw strongly on critical thinking. In keeping with prior analyses ( Reynolds and Thompson, 2011 ; Dowd et al. , 2016 ), these findings further reinforce the notion that disciplinary instructors, who are most capable of teaching and assessing scientific reasoning and perhaps least interested in the more mechanical aspects of writing, may nonetheless be best suited to effectively model and assess students’ writing.
The goal of the thesis writing course at both Duke University and the University of Minnesota is not merely to improve thesis scores but to move students’ writing into the category of mastery across BioTAP dimensions. Recognizing that students with differing critical-thinking skills (particularly inference) are more or less likely to achieve mastery in the undergraduate thesis (particularly in discussing implications [question 5]) is important for developing and testing targeted pedagogical interventions to improve learning outcomes for all students.
The competencies characterized by the Vision and Change in Undergraduate Biology Education Initiative provide a general framework for recognizing that science reasoning and critical-thinking skills play key roles in major learning outcomes of science education. Our findings highlight places where science reasoning–related competencies (like “understanding the process of science”) connect to critical-thinking skills and places where critical thinking–related competencies might be manifested in scientific products (such as the ability to discuss implications in scientific writing). We encourage broader efforts to build empirical connections between competencies and pedagogical practices to further improve science education.
One specific implication of this work for science education is to focus on providing opportunities for students to develop their critical-thinking skills (particularly inference). Of course, as this correlational study is not designed to test causality, we do not claim that enhancing students’ inference skills will improve science reasoning in writing. However, as prior work shows that science writing activities influence students’ inference skills ( Quitadamo and Kurtz, 2007 ; Quitadamo et al. , 2008 ), there is reason to test such a hypothesis. Nevertheless, the focus must extend beyond inference as an isolated skill; rather, it is important to relate inference to the foundations of the scientific method ( Miri et al. , 2007 ) in terms of the epistemological appreciation of the functions and coordination of evidence ( Kuhn and Dean, 2004 ; Zeineddin and Abd-El-Khalick, 2010 ; Ding et al. , 2016 ) and disciplinary paradigms of truth and justification ( Moshman, 2015 ).
Although this study is limited to the domain of biology at two institutions with a relatively small number of students, the findings represent a foundational step in the direction of achieving success with more integrated learning outcomes. Hopefully, it will spur greater interest in empirically grounding discussions of the constructs of scientific reasoning and critical-thinking skills.
This study contributes to the efforts to improve science education, for both majors and nonmajors, through an empirically driven analysis of the relationships between scientific reasoning reflected in the genre of thesis writing and critical-thinking skills. This work is rooted in the usefulness of BioTAP as a method 1) to facilitate communication and learning and 2) to assess disciplinary-specific and general dimensions of science reasoning. The findings support the important role of the critical-thinking skill of inference in scientific reasoning in writing, while also highlighting ways in which other aspects of science reasoning (epistemological considerations, writing conventions, etc.) are not significantly related to critical thinking. Future research into the impact of interventions focused on specific critical-thinking skills (i.e., inference) for improved science reasoning in writing will build on this work and its implications for science education.
Supplementary Material
Acknowledgments.
We acknowledge the contributions of Kelaine Haas and Alexander Motten to the implementation and collection of data. We also thank Mine Çetinkaya-Rundel for her insights regarding our statistical analyses. This research was funded by National Science Foundation award DUE-1525602.
- American Association for the Advancement of Science. (2011). Vision and change in undergraduate biology education: A call to action . Washington, DC: Retrieved September 26, 2017, from https://visionandchange.org/files/2013/11/aaas-VISchange-web1113.pdf . [ Google Scholar ]
- August D. (2016). California Critical Thinking Skills Test user manual and resource guide . San Jose: Insight Assessment/California Academic Press. [ Google Scholar ]
- Beyer C. H., Taylor E., Gillmore G. M. (2013). Inside the undergraduate teaching experience: The University of Washington’s growth in faculty teaching study . Albany, NY: SUNY Press. [ Google Scholar ]
- Bissell A. N., Lemons P. P. (2006). A new method for assessing critical thinking in the classroom . BioScience , ( 1 ), 66–72. https://doi.org/10.1641/0006-3568(2006)056[0066:ANMFAC]2.0.CO;2 . [ Google Scholar ]
- Blattner N. H., Frazier C. L. (2002). Developing a performance-based assessment of students’ critical thinking skills . Assessing Writing , ( 1 ), 47–64. [ Google Scholar ]
- Clase K. L., Gundlach E., Pelaez N. J. (2010). Calibrated peer review for computer-assisted learning of biological research competencies . Biochemistry and Molecular Biology Education , ( 5 ), 290–295. [ PubMed ] [ Google Scholar ]
- Condon W., Kelly-Riley D. (2004). Assessing and teaching what we value: The relationship between college-level writing and critical thinking abilities . Assessing Writing , ( 1 ), 56–75. https://doi.org/10.1016/j.asw.2004.01.003 . [ Google Scholar ]
- Ding L., Wei X., Liu X. (2016). Variations in university students’ scientific reasoning skills across majors, years, and types of institutions . Research in Science Education , ( 5 ), 613–632. https://doi.org/10.1007/s11165-015-9473-y . [ Google Scholar ]
- Dowd J. E., Connolly M. P., Thompson R. J., Jr., Reynolds J. A. (2015a). Improved reasoning in undergraduate writing through structured workshops . Journal of Economic Education , ( 1 ), 14–27. https://doi.org/10.1080/00220485.2014.978924 . [ Google Scholar ]
- Dowd J. E., Roy C. P., Thompson R. J., Jr., Reynolds J. A. (2015b). “On course” for supporting expanded participation and improving scientific reasoning in undergraduate thesis writing . Journal of Chemical Education , ( 1 ), 39–45. https://doi.org/10.1021/ed500298r . [ Google Scholar ]
- Dowd J. E., Thompson R. J., Jr., Reynolds J. A. (2016). Quantitative genre analysis of undergraduate theses: Uncovering different ways of writing and thinking in science disciplines . WAC Journal , , 36–51. [ Google Scholar ]
- Facione P. A. (1990). Critical thinking: a statement of expert consensus for purposes of educational assessment and instruction. Research findings and recommendations . Newark, DE: American Philosophical Association; Retrieved September 26, 2017, from https://philpapers.org/archive/FACCTA.pdf . [ Google Scholar ]
- Gerdeman R. D., Russell A. A., Worden K. J., Gerdeman R. D., Russell A. A., Worden K. J. (2007). Web-based student writing and reviewing in a large biology lecture course . Journal of College Science Teaching , ( 5 ), 46–52. [ Google Scholar ]
- Greenhoot A. F., Semb G., Colombo J., Schreiber T. (2004). Prior beliefs and methodological concepts in scientific reasoning . Applied Cognitive Psychology , ( 2 ), 203–221. https://doi.org/10.1002/acp.959 . [ Google Scholar ]
- Haaga D. A. F. (1993). Peer review of term papers in graduate psychology courses . Teaching of Psychology , ( 1 ), 28–32. https://doi.org/10.1207/s15328023top2001_5 . [ Google Scholar ]
- Halonen J. S., Bosack T., Clay S., McCarthy M., Dunn D. S., Hill G. W., Whitlock K. (2003). A rubric for learning, teaching, and assessing scientific inquiry in psychology . Teaching of Psychology , ( 3 ), 196–208. https://doi.org/10.1207/S15328023TOP3003_01 . [ Google Scholar ]
- Hand B., Keys C. W. (1999). Inquiry investigation . Science Teacher , ( 4 ), 27–29. [ Google Scholar ]
- Holm S. (1979). A simple sequentially rejective multiple test procedure . Scandinavian Journal of Statistics , ( 2 ), 65–70. [ Google Scholar ]
- Holyoak K. J., Morrison R. G. (2005). The Cambridge handbook of thinking and reasoning . New York: Cambridge University Press. [ Google Scholar ]
- Insight Assessment. (2016a). California Critical Thinking Skills Test (CCTST) Retrieved September 26, 2017, from www.insightassessment.com/Products/Products-Summary/Critical-Thinking-Skills-Tests/California-Critical-Thinking-Skills-Test-CCTST .
- Insight Assessment. (2016b). Sample thinking skills questions. Retrieved September 26, 2017, from www.insightassessment.com/Resources/Teaching-Training-and-Learning-Tools/node_1487 .
- Kelly G. J., Takao A. (2002). Epistemic levels in argument: An analysis of university oceanography students’ use of evidence in writing . Science Education , ( 3 ), 314–342. https://doi.org/10.1002/sce.10024 . [ Google Scholar ]
- Kuhn D., Dean D., Jr. (2004). Connecting scientific reasoning and causal inference . Journal of Cognition and Development , ( 2 ), 261–288. https://doi.org/10.1207/s15327647jcd0502_5 . [ Google Scholar ]
- Kuhn D., Iordanou K., Pease M., Wirkala C. (2008). Beyond control of variables: What needs to develop to achieve skilled scientific thinking? . Cognitive Development , ( 4 ), 435–451. https://doi.org/10.1016/j.cogdev.2008.09.006 . [ Google Scholar ]
- Lawson A. E. (2010). Basic inferences of scientific reasoning, argumentation, and discovery . Science Education , ( 2 ), 336–364. https://doi.org/10.1002/sce.20357 . [ Google Scholar ]
- Meizlish D., LaVaque-Manty D., Silver N., Kaplan M. (2013). Think like/write like: Metacognitive strategies to foster students’ development as disciplinary thinkers and writers . In Thompson R. J. (Ed.), Changing the conversation about higher education (pp. 53–73). Lanham, MD: Rowman & Littlefield. [ Google Scholar ]
- Miri B., David B.-C., Uri Z. (2007). Purposely teaching for the promotion of higher-order thinking skills: A case of critical thinking . Research in Science Education , ( 4 ), 353–369. https://doi.org/10.1007/s11165-006-9029-2 . [ Google Scholar ]
- Moshman D. (2015). Epistemic cognition and development: The psychology of justification and truth . New York: Psychology Press. [ Google Scholar ]
- National Research Council. (2000). How people learn: Brain, mind, experience, and school . Expanded ed. Washington, DC: National Academies Press. [ Google Scholar ]
- Pukkila P. J. (2004). Introducing student inquiry in large introductory genetics classes . Genetics , ( 1 ), 11–18. https://doi.org/10.1534/genetics.166.1.11 . [ PMC free article ] [ PubMed ] [ Google Scholar ]
- Quitadamo I. J., Faiola C. L., Johnson J. E., Kurtz M. J. (2008). Community-based inquiry improves critical thinking in general education biology . CBE—Life Sciences Education , ( 3 ), 327–337. https://doi.org/10.1187/cbe.07-11-0097 . [ PMC free article ] [ PubMed ] [ Google Scholar ]
- Quitadamo I. J., Kurtz M. J. (2007). Learning to improve: Using writing to increase critical thinking performance in general education biology . CBE—Life Sciences Education , ( 2 ), 140–154. https://doi.org/10.1187/cbe.06-11-0203 . [ PMC free article ] [ PubMed ] [ Google Scholar ]
- Reynolds J. A., Smith R., Moskovitz C., Sayle A. (2009). BioTAP: A systematic approach to teaching scientific writing and evaluating undergraduate theses . BioScience , ( 10 ), 896–903. https://doi.org/10.1525/bio.2009.59.10.11 . [ Google Scholar ]
- Reynolds J. A., Thaiss C., Katkin W., Thompson R. J. (2012). Writing-to-learn in undergraduate science education: A community-based, conceptually driven approach . CBE—Life Sciences Education , ( 1 ), 17–25. https://doi.org/10.1187/cbe.11-08-0064 . [ PMC free article ] [ PubMed ] [ Google Scholar ]
- Reynolds J. A., Thompson R. J. (2011). Want to improve undergraduate thesis writing? Engage students and their faculty readers in scientific peer review . CBE—Life Sciences Education , ( 2 ), 209–215. https://doi.org/10.1187/cbe.10-10-0127 . [ PMC free article ] [ PubMed ] [ Google Scholar ]
- Rhemtulla M., Brosseau-Liard P. E., Savalei V. (2012). When can categorical variables be treated as continuous? A comparison of robust continuous and categorical SEM estimation methods under suboptimal conditions . Psychological Methods , ( 3 ), 354–373. https://doi.org/10.1037/a0029315 . [ PubMed ] [ Google Scholar ]
- Stephenson N. S., Sadler-McKnight N. P. (2016). Developing critical thinking skills using the science writing heuristic in the chemistry laboratory . Chemistry Education Research and Practice , ( 1 ), 72–79. https://doi.org/10.1039/C5RP00102A . [ Google Scholar ]
- Tariq V. N., Stefani L. A. J., Butcher A. C., Heylings D. J. A. (1998). Developing a new approach to the assessment of project work . Assessment and Evaluation in Higher Education , ( 3 ), 221–240. https://doi.org/10.1080/0260293980230301 . [ Google Scholar ]
- Timmerman B. E. C., Strickland D. C., Johnson R. L., Payne J. R. (2011). Development of a “universal” rubric for assessing undergraduates’ scientific reasoning skills using scientific writing . Assessment and Evaluation in Higher Education , ( 5 ), 509–547. https://doi.org/10.1080/02602930903540991 . [ Google Scholar ]
- Topping K. J., Smith E. F., Swanson I., Elliot A. (2000). Formative peer assessment of academic writing between postgraduate students . Assessment and Evaluation in Higher Education , ( 2 ), 149–169. https://doi.org/10.1080/713611428 . [ Google Scholar ]
- Willison J., O’Regan K. (2007). Commonly known, commonly not known, totally unknown: A framework for students becoming researchers . Higher Education Research and Development , ( 4 ), 393–409. https://doi.org/10.1080/07294360701658609 . [ Google Scholar ]
- Woodin T., Carter V. C., Fletcher L. (2010). Vision and Change in Biology Undergraduate Education: A Call for Action—Initial responses . CBE—Life Sciences Education , ( 2 ), 71–73. https://doi.org/10.1187/cbe.10-03-0044 . [ PMC free article ] [ PubMed ] [ Google Scholar ]
- Zeineddin A., Abd-El-Khalick F. (2010). Scientific reasoning and epistemological commitments: Coordination of theory and evidence among college science students . Journal of Research in Science Teaching , ( 9 ), 1064–1093. https://doi.org/10.1002/tea.20368 . [ Google Scholar ]
- Zimmerman C. (2000). The development of scientific reasoning skills . Developmental Review , ( 1 ), 99–149. https://doi.org/10.1006/drev.1999.0497 . [ Google Scholar ]
- Zimmerman C. (2007). The development of scientific thinking skills in elementary and middle school . Developmental Review , ( 2 ), 172–223. https://doi.org/10.1016/j.dr.2006.12.001 . [ Google Scholar ]
- Corpus ID: 67223434
The Acquisition of Scientific Knowledge Via Critical Thinking: A Philosophical Approach to Science Education
- I. Talavera
- Published 22 June 2016
- Philosophy, Education
One Citation
Learning science: physical and life sciences in curricula across u.s. schools of nursing, 64 references, conceptualizations of argumentation from science studies and the learning sciences and their implications for the practices of science education, science and religion: drawing the line, psychic powers, astrology & creationism in the classroom evidence of pseudoscientific beliefs among high school biology & life science teachers, introduction: what is the philosophy of science, teaching evolution (and all of biology) more effectively: strategies for engagement, critical reasoning, and confronting misconceptions., beyond ethical codes: a call for critical thinking in religious culture, science for all americans, the effect of engaging prior learning on student attitudes toward creationism and evolution, unintended consequences: how science professors discourage women of color, is knowledge of science associated with higher skepticism of pseudoscientific claims, related papers.
Showing 1 through 3 of 0 Related Papers

- Table of Contents
- Random Entry
- Chronological
- Editorial Information
- About the SEP
- Editorial Board
- How to Cite the SEP
- Special Characters
- Advanced Tools
- Support the SEP
- PDFs for SEP Friends
- Make a Donation
- SEPIA for Libraries
- Back to Entry
- Entry Contents
- Entry Bibliography
- Academic Tools
- Friends PDF Preview
- Author and Citation Info
- Back to Top
Supplement to Critical Thinking
This supplement elaborates on the history of the articulation, promotion and adoption of critical thinking as an educational goal.
John Dewey (1910: 74, 82) introduced the term ‘critical thinking’ as the name of an educational goal, which he identified with a scientific attitude of mind. More commonly, he called the goal ‘reflective thought’, ‘reflective thinking’, ‘reflection’, or just ‘thought’ or ‘thinking’. He describes his book as written for two purposes. The first was to help people to appreciate the kinship of children’s native curiosity, fertile imagination and love of experimental inquiry to the scientific attitude. The second was to help people to consider how recognizing this kinship in educational practice “would make for individual happiness and the reduction of social waste” (iii). He notes that the ideas in the book obtained concreteness in the Laboratory School in Chicago.
Dewey’s ideas were put into practice by some of the schools that participated in the Eight-Year Study in the 1930s sponsored by the Progressive Education Association in the United States. For this study, 300 colleges agreed to consider for admission graduates of 30 selected secondary schools or school systems from around the country who experimented with the content and methods of teaching, even if the graduates had not completed the then-prescribed secondary school curriculum. One purpose of the study was to discover through exploration and experimentation how secondary schools in the United States could serve youth more effectively (Aikin 1942). Each experimental school was free to change the curriculum as it saw fit, but the schools agreed that teaching methods and the life of the school should conform to the idea (previously advocated by Dewey) that people develop through doing things that are meaningful to them, and that the main purpose of the secondary school was to lead young people to understand, appreciate and live the democratic way of life characteristic of the United States (Aikin 1942: 17–18). In particular, school officials believed that young people in a democracy should develop the habit of reflective thinking and skill in solving problems (Aikin 1942: 81). Students’ work in the classroom thus consisted more often of a problem to be solved than a lesson to be learned. Especially in mathematics and science, the schools made a point of giving students experience in clear, logical thinking as they solved problems. The report of one experimental school, the University School of Ohio State University, articulated this goal of improving students’ thinking:
Critical or reflective thinking originates with the sensing of a problem. It is a quality of thought operating in an effort to solve the problem and to reach a tentative conclusion which is supported by all available data. It is really a process of problem solving requiring the use of creative insight, intellectual honesty, and sound judgment. It is the basis of the method of scientific inquiry. The success of democracy depends to a large extent on the disposition and ability of citizens to think critically and reflectively about the problems which must of necessity confront them, and to improve the quality of their thinking is one of the major goals of education. (Commission on the Relation of School and College of the Progressive Education Association 1943: 745–746)
The Eight-Year Study had an evaluation staff, which developed, in consultation with the schools, tests to measure aspects of student progress that fell outside the focus of the traditional curriculum. The evaluation staff classified many of the schools’ stated objectives under the generic heading “clear thinking” or “critical thinking” (Smith, Tyler, & Evaluation Staff 1942: 35–36). To develop tests of achievement of this broad goal, they distinguished five overlapping aspects of it: ability to interpret data, abilities associated with an understanding of the nature of proof, and the abilities to apply principles of science, of social studies and of logical reasoning. The Eight-Year Study also had a college staff, directed by a committee of college administrators, whose task was to determine how well the experimental schools had prepared their graduates for college. The college staff compared the performance of 1,475 college students from the experimental schools with an equal number of graduates from conventional schools, matched in pairs by sex, age, race, scholastic aptitude scores, home and community background, interests, and probable future. They concluded that, on 18 measures of student success, the graduates of the experimental schools did a somewhat better job than the comparison group. The graduates from the six most traditional of the experimental schools showed no large or consistent differences. The graduates from the six most experimental schools, on the other hand, had much greater differences in their favour. The graduates of the two most experimental schools, the college staff reported:
… surpassed their comparison groups by wide margins in academic achievement, intellectual curiosity, scientific approach to problems, and interest in contemporary affairs. The differences in their favor were even greater in general resourcefulness, in enjoyment of reading, [in] participation in the arts, in winning non-academic honors, and in all aspects of college life except possibly participation in sports and social activities. (Aikin 1942: 114)
One of these schools was a private school with students from privileged families and the other the experimental section of a public school with students from non-privileged families. The college staff reported that the graduates of the two schools were indistinguishable from each other in terms of college success.
In 1933 Dewey issued an extensively rewritten edition of his How We Think (Dewey 1910), with the sub-title “A restatement of the relation of reflective thinking to the educative process”. Although the restatement retains the basic structure and content of the original book, Dewey made a number of changes. He rewrote and simplified his logical analysis of the process of reflection, made his ideas clearer and more definite, replaced the terms ‘induction’ and ‘deduction’ by the phrases ‘control of data and evidence’ and ‘control of reasoning and concepts’, added more illustrations, rearranged chapters, and revised the parts on teaching to reflect changes in schools since 1910. In particular, he objected to one-sided practices of some “experimental” and “progressive” schools that allowed children freedom but gave them no guidance, citing as objectionable practices novelty and variety for their own sake, experiences and activities with real materials but of no educational significance, treating random and disconnected activity as if it were an experiment, failure to summarize net accomplishment at the end of an inquiry, non-educative projects, and treatment of the teacher as a negligible factor rather than as “the intellectual leader of a social group” (Dewey 1933: 273). Without explaining his reasons, Dewey eliminated the previous edition’s uses of the words ‘critical’ and ‘uncritical’, thus settling firmly on ‘reflection’ or ‘reflective thinking’ as the preferred term for his subject-matter. In the revised edition, the word ‘critical’ occurs only once, where Dewey writes that “a person may not be sufficiently critical about the ideas that occur to him” (1933: 16, italics in original); being critical is thus a component of reflection, not the whole of it. In contrast, the Eight-Year Study by the Progressive Education Association treated ‘critical thinking’ and ‘reflective thinking’ as synonyms.
In the same period, Dewey collaborated on a history of the Laboratory School in Chicago with two former teachers from the school (Mayhew & Edwards 1936). The history describes the school’s curriculum and organization, activities aimed at developing skills, parents’ involvement, and the habits of mind that the children acquired. A concluding chapter evaluates the school’s achievements, counting as a success its staging of the curriculum to correspond to the natural development of the growing child. In two appendices, the authors describe the evolution of Dewey’s principles of education and Dewey himself describes the theory of the Chicago experiment (Dewey 1936).
Glaser (1941) reports in his doctoral dissertation the method and results of an experiment in the development of critical thinking conducted in the fall of 1938. He defines critical thinking as Dewey defined reflective thinking:
Critical thinking calls for a persistent effort to examine any belief or supposed form of knowledge in the light of the evidence that supports it and the further conclusions to which it tends. (Glaser 1941: 6; cf. Dewey 1910: 6; Dewey 1933: 9)
In the experiment, eight lesson units directed at improving critical thinking abilities were taught to four grade 12 high school classes, with pre-test and post-test of the students using the Otis Quick-Scoring Mental Ability Test and the Watson-Glaser Tests of Critical Thinking (developed in collaboration with Glaser’s dissertation sponsor, Goodwin Watson). The average gain in scores on these tests was greater to a statistically significant degree among the students who received the lessons in critical thinking than among the students in a control group of four grade 12 high school classes taking the usual curriculum in English. Glaser concludes:
The aspect of critical thinking which appears most susceptible to general improvement is the attitude of being disposed to consider in a thoughtful way the problems and subjects that come within the range of one’s experience. An attitude of wanting evidence for beliefs is more subject to general transfer. Development of skill in applying the methods of logical inquiry and reasoning, however, appears to be specifically related to, and in fact limited by, the acquisition of pertinent knowledge and facts concerning the problem or subject matter toward which the thinking is to be directed. (Glaser 1941: 175)
Retest scores and observable behaviour indicated that students in the intervention group retained their growth in ability to think critically for at least six months after the special instruction.
In 1948 a group of U.S. college examiners decided to develop taxonomies of educational objectives with a common vocabulary that they could use for communicating with each other about test items. The first of these taxonomies, for the cognitive domain, appeared in 1956 (Bloom et al. 1956), and included critical thinking objectives. It has become known as Bloom’s taxonomy. A second taxonomy, for the affective domain (Krathwohl, Bloom, & Masia 1964), and a third taxonomy, for the psychomotor domain (Simpson 1966–67), appeared later. Each of the taxonomies is hierarchical, with achievement of a higher educational objective alleged to require achievement of corresponding lower educational objectives.
Bloom’s taxonomy has six major categories. From lowest to highest, they are knowledge, comprehension, application, analysis, synthesis, and evaluation. Within each category, there are sub-categories, also arranged hierarchically from the educationally prior to the educationally posterior. The lowest category, though called ‘knowledge’, is confined to objectives of remembering information and being able to recall or recognize it, without much transformation beyond organizing it (Bloom et al. 1956: 28–29). The five higher categories are collectively termed “intellectual abilities and skills” (Bloom et al. 1956: 204). The term is simply another name for critical thinking abilities and skills:
Although information or knowledge is recognized as an important outcome of education, very few teachers would be satisfied to regard this as the primary or the sole outcome of instruction. What is needed is some evidence that the students can do something with their knowledge, that is, that they can apply the information to new situations and problems. It is also expected that students will acquire generalized techniques for dealing with new problems and new materials. Thus, it is expected that when the student encounters a new problem or situation, he will select an appropriate technique for attacking it and will bring to bear the necessary information, both facts and principles. This has been labeled “critical thinking” by some, “reflective thinking” by Dewey and others, and “problem solving” by still others. In the taxonomy, we have used the term “intellectual abilities and skills”. (Bloom et al. 1956: 38)
Comprehension and application objectives, as their names imply, involve understanding and applying information. Critical thinking abilities and skills show up in the three highest categories of analysis, synthesis and evaluation. The condensed version of Bloom’s taxonomy (Bloom et al. 1956: 201–207) gives the following examples of objectives at these levels:
- analysis objectives : ability to recognize unstated assumptions, ability to check the consistency of hypotheses with given information and assumptions, ability to recognize the general techniques used in advertising, propaganda and other persuasive materials
- synthesis objectives : organizing ideas and statements in writing, ability to propose ways of testing a hypothesis, ability to formulate and modify hypotheses
- evaluation objectives : ability to indicate logical fallacies, comparison of major theories about particular cultures
The analysis, synthesis and evaluation objectives in Bloom’s taxonomy collectively came to be called the “higher-order thinking skills” (Tankersley 2005: chap. 5). Although the analysis-synthesis-evaluation sequence mimics phases in Dewey’s (1933) logical analysis of the reflective thinking process, it has not generally been adopted as a model of a critical thinking process. While commending the inspirational value of its ratio of five categories of thinking objectives to one category of recall objectives, Ennis (1981b) points out that the categories lack criteria applicable across topics and domains. For example, analysis in chemistry is so different from analysis in literature that there is not much point in teaching analysis as a general type of thinking. Further, the postulated hierarchy seems questionable at the higher levels of Bloom’s taxonomy. For example, ability to indicate logical fallacies hardly seems more complex than the ability to organize statements and ideas in writing.
A revised version of Bloom’s taxonomy (Anderson et al. 2001) distinguishes the intended cognitive process in an educational objective (such as being able to recall, to compare or to check) from the objective’s informational content (“knowledge”), which may be factual, conceptual, procedural, or metacognitive. The result is a so-called “Taxonomy Table” with four rows for the kinds of informational content and six columns for the six main types of cognitive process. The authors name the types of cognitive process by verbs, to indicate their status as mental activities. They change the name of the ‘comprehension’ category to ‘understand’ and of the ‘synthesis’ category to ’create’, and switch the order of synthesis and evaluation. The result is a list of six main types of cognitive process aimed at by teachers: remember, understand, apply, analyze, evaluate, and create. The authors retain the idea of a hierarchy of increasing complexity, but acknowledge some overlap, for example between understanding and applying. And they retain the idea that critical thinking and problem solving cut across the more complex cognitive processes. The terms ‘critical thinking’ and ‘problem solving’, they write:
are widely used and tend to become touchstones of curriculum emphasis. Both generally include a variety of activities that might be classified in disparate cells of the Taxonomy Table. That is, in any given instance, objectives that involve problem solving and critical thinking most likely call for cognitive processes in several categories on the process dimension. For example, to think critically about an issue probably involves some Conceptual knowledge to Analyze the issue. Then, one can Evaluate different perspectives in terms of the criteria and, perhaps, Create a novel, yet defensible perspective on this issue. (Anderson et al. 2001: 269–270; italics in original)
In the revised taxonomy, only a few sub-categories, such as inferring, have enough commonality to be treated as a distinct critical thinking ability that could be taught and assessed as a general ability.
A landmark contribution to philosophical scholarship on the concept of critical thinking was a 1962 article in the Harvard Educational Review by Robert H. Ennis, with the title “A concept of critical thinking: A proposed basis for research in the teaching and evaluation of critical thinking ability” (Ennis 1962). Ennis took as his starting-point a conception of critical thinking put forward by B. Othanel Smith:
We shall consider thinking in terms of the operations involved in the examination of statements which we, or others, may believe. A speaker declares, for example, that “Freedom means that the decisions in America’s productive effort are made not in the minds of a bureaucracy but in the free market”. Now if we set about to find out what this statement means and to determine whether to accept or reject it, we would be engaged in thinking which, for lack of a better term, we shall call critical thinking. If one wishes to say that this is only a form of problem-solving in which the purpose is to decide whether or not what is said is dependable, we shall not object. But for our purposes we choose to call it critical thinking. (Smith 1953: 130)
Adding a normative component to this conception, Ennis defined critical thinking as “the correct assessing of statements” (Ennis 1962: 83). On the basis of this definition, he distinguished 12 “aspects” of critical thinking corresponding to types or aspects of statements, such as judging whether an observation statement is reliable and grasping the meaning of a statement. He noted that he did not include judging value statements. Cutting across the 12 aspects, he distinguished three dimensions of critical thinking: logical (judging relationships between meanings of words and statements), criterial (knowledge of the criteria for judging statements), and pragmatic (the impression of the background purpose). For each aspect, Ennis described the applicable dimensions, including criteria. He proposed the resulting construct as a basis for developing specifications for critical thinking tests and for research on instructional methods and levels.
In the 1970s and 1980s there was an upsurge of attention to the development of thinking skills. The annual International Conference on Critical Thinking and Educational Reform has attracted since its start in 1980 tens of thousands of educators from all levels. In 1983 the College Entrance Examination Board proclaimed reasoning as one of six basic academic competencies needed by college students (College Board 1983). Departments of education in the United States and around the world began to include thinking objectives in their curriculum guidelines for school subjects. For example, Ontario’s social sciences and humanities curriculum guideline for secondary schools requires “the use of critical and creative thinking skills and/or processes” as a goal of instruction and assessment in each subject and course (Ontario Ministry of Education 2013: 30). The document describes critical thinking as follows:
Critical thinking is the process of thinking about ideas or situations in order to understand them fully, identify their implications, make a judgement, and/or guide decision making. Critical thinking includes skills such as questioning, predicting, analysing, synthesizing, examining opinions, identifying values and issues, detecting bias, and distinguishing between alternatives. Students who are taught these skills become critical thinkers who can move beyond superficial conclusions to a deeper understanding of the issues they are examining. They are able to engage in an inquiry process in which they explore complex and multifaceted issues, and questions for which there may be no clear-cut answers (Ontario Ministry of Education 2013: 46).
Sweden makes schools responsible for ensuring that each pupil who completes compulsory school “can make use of critical thinking and independently formulate standpoints based on knowledge and ethical considerations” (Skolverket 2018: 12). Subject syllabi incorporate this requirement, and items testing critical thinking skills appear on national tests that are a required step toward university admission. For example, the core content of biology, physics and chemistry in years 7-9 includes critical examination of sources of information and arguments encountered by pupils in different sources and social discussions related to these sciences, in both digital and other media. (Skolverket 2018: 170, 181, 192). Correspondingly, in year 9 the national tests require using knowledge of biology, physics or chemistry “to investigate information, communicate and come to a decision on issues concerning health, energy, technology, the environment, use of natural resources and ecological sustainability” (see the message from the School Board ). Other jurisdictions similarly embed critical thinking objectives in curriculum guidelines.
At the college level, a new wave of introductory logic textbooks, pioneered by Kahane (1971), applied the tools of logic to contemporary social and political issues. Popular contemporary textbooks of this sort include those by Bailin and Battersby (2016b), Boardman, Cavender and Kahane (2018), Browne and Keeley (2018), Groarke and Tindale (2012), and Moore and Parker (2020). In their wake, colleges and universities in North America transformed their introductory logic course into a general education service course with a title like ‘critical thinking’ or ‘reasoning’. In 1980, the trustees of California’s state university and colleges approved as a general education requirement a course in critical thinking, described as follows:
Instruction in critical thinking is to be designed to achieve an understanding of the relationship of language to logic, which should lead to the ability to analyze, criticize, and advocate ideas, to reason inductively and deductively, and to reach factual or judgmental conclusions based on sound inferences drawn from unambiguous statements of knowledge or belief. The minimal competence to be expected at the successful conclusion of instruction in critical thinking should be the ability to distinguish fact from judgment, belief from knowledge, and skills in elementary inductive and deductive processes, including an understanding of the formal and informal fallacies of language and thought. (Dumke 1980)
Since December 1983, the Association for Informal Logic and Critical Thinking has sponsored sessions at the three annual divisional meetings of the American Philosophical Association. In December 1987, the Committee on Pre-College Philosophy of the American Philosophical Association invited Peter Facione to make a systematic inquiry into the current state of critical thinking and critical thinking assessment. Facione assembled a group of 46 other academic philosophers and psychologists to participate in a multi-round Delphi process, whose product was entitled Critical Thinking: A Statement of Expert Consensus for Purposes of Educational Assessment and Instruction (Facione 1990a). The statement listed abilities and dispositions that should be the goals of a lower-level undergraduate course in critical thinking. Researchers in nine European countries determined which of these skills and dispositions employers expect of university graduates (Dominguez 2018 a), compared those expectations to critical thinking educational practices in post-secondary educational institutions (Dominguez 2018b), developed a course on critical thinking education for university teachers (Dominguez 2018c) and proposed in response to identified gaps between expectations and practices an “educational protocol” that post-secondary educational institutions in Europe could use to develop critical thinking (Elen et al. 2019).
Copyright © 2022 by David Hitchcock < hitchckd @ mcmaster . ca >
- Accessibility
Support SEP
Mirror sites.
View this site from another server:
- Info about mirror sites
The Stanford Encyclopedia of Philosophy is copyright © 2024 by The Metaphysics Research Lab , Department of Philosophy, Stanford University
Library of Congress Catalog Data: ISSN 1095-5054
- February 12, 2024
The Vital Role of Critical Thinking in Knowledge Acquisition

In the ever-evolving landscape of education and professional development, the ability to sift through vast amounts of information and distil it into actionable knowledge is more critical than ever. At the heart of this transformation lies a skill often heralded yet not deeply explored: critical thinking.
It’s a term frequently mentioned in academic and professional circles, but what does it truly entail, and more importantly, why is it indispensable in our journey from gathering information to acquiring knowledge?
Critical thinking is not just a supplementary skill; it’s the linchpin that converts raw data and facts into meaningful understanding and insight. It’s the process of actively analyzing, synthesizing, and evaluating information gathered from observation, experience, reflection, or communication.
In an age where information is ubiquitous, the ability to critically examine this information and apply reasoned judgment is what distinguishes mere data holders from true knowledge seekers.
This ability to think critically does not merely enhance knowledge acquisition; it fundamentally reshapes it. Instead of passively receiving information, critical thinkers engage actively with content, questioning assumptions, seeking evidence, and drawing connections. This dynamic interaction with information is what transforms it into knowledge – a process far more profound than simple memorization or passive consumption.
Thus, the main argument of this discussion is clear: critical thinking is not just an adjunct but a central component of effective knowledge acquisition. It’s the bridge between having access to information and being able to understand, interpret, and apply that information effectively in various contexts – a skill crucial not only for academic success but also for navigating the complexities of today’s professional environments.
In the following sections, we will delve deeper into the mechanics of critical thinking, explore its role in the process of learning, and provide practical insights on how to cultivate this essential skill, thus empowering our journey from information-rich environments to knowledge-empowered landscapes.
Understanding Critical Thinking
At its core, critical thinking is a disciplined process of thought that enhances our ability to interpret, analyze, and evaluate information and situations.
It’s a multifaceted skill, encompassing several key elements that are essential in various aspects of life, especially in the realm of knowledge acquisition.
Definition and Key Elements
Critical thinking involves a range of cognitive skills and dispositions.
Here are some of the pivotal elements:
- Analysis : This involves breaking down complex pieces of information or problems into smaller, more manageable parts to understand them better.
- Evaluation : Critical thinkers assess the credibility and logical strength of evidence, arguments, and claims. They discern bias, identify inconsistencies, and evaluate the validity of sources and content.
- Inference : The ability to draw reasoned conclusions from the information at hand is a crucial aspect of critical thinking. It involves seeing beyond the obvious and making connections between seemingly unrelated pieces of information.
- Problem-Solving : This skill is about using critical analysis, creative thinking, and logical reasoning to find solutions to complex problems. It’s about thinking beyond the standard approaches and considering a range of potential solutions.
- Reflection : Critical thinking involves a degree of self-reflection, questioning one’s own assumptions, beliefs, and values, and considering how these might influence one’s interpretations and judgments.
The Relationship Between Critical Thinking and Knowledge Acquisition
The relationship between critical thinking and knowledge acquisition is profound and integral.
Here’s how these skills contribute to effective knowledge acquisition:
- Enhanced Comprehension : By analysing and breaking down information, critical thinkers gain a deeper understanding of the subject matter, moving beyond surface-level comprehension to a more nuanced grasp.
- Effective Application : Evaluation and inference skills allow individuals to apply information in new and diverse contexts. Knowledge isn’t just about storing information; it’s about using it effectively to make decisions, solve problems, and generate new ideas.
- Informed Decision-Making : Through critical evaluation, individuals can make well-informed decisions, discerning the most relevant and accurate information amidst a sea of data.
- Continuous Learning : The reflective aspect of critical thinking fosters a mindset of continuous learning . Critical thinkers are always questioning, always learning, and perpetually refining their understanding of the world around them.
In summary, critical thinking is not just a supplementary skill but a cornerstone in the process of transforming information into meaningful and applicable knowledge.
It is through these critical lenses that we not only view and interpret the world but also contribute to it with informed insights and innovative solutions.
Chris Hutchinson
Share this post, more to explore.
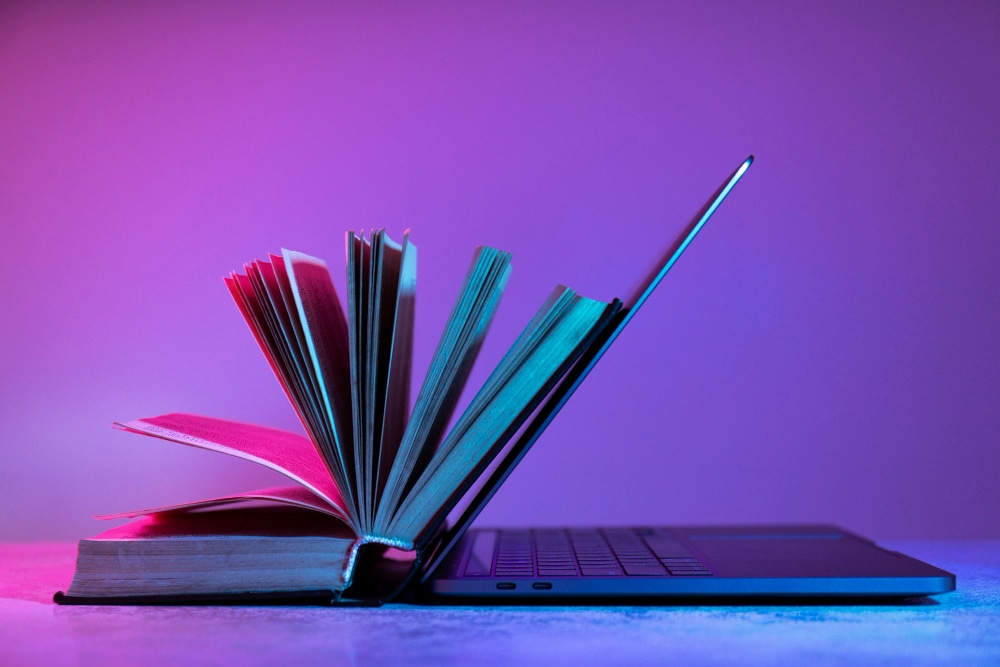
Claned has been chosen one of the top edtech companies in the world
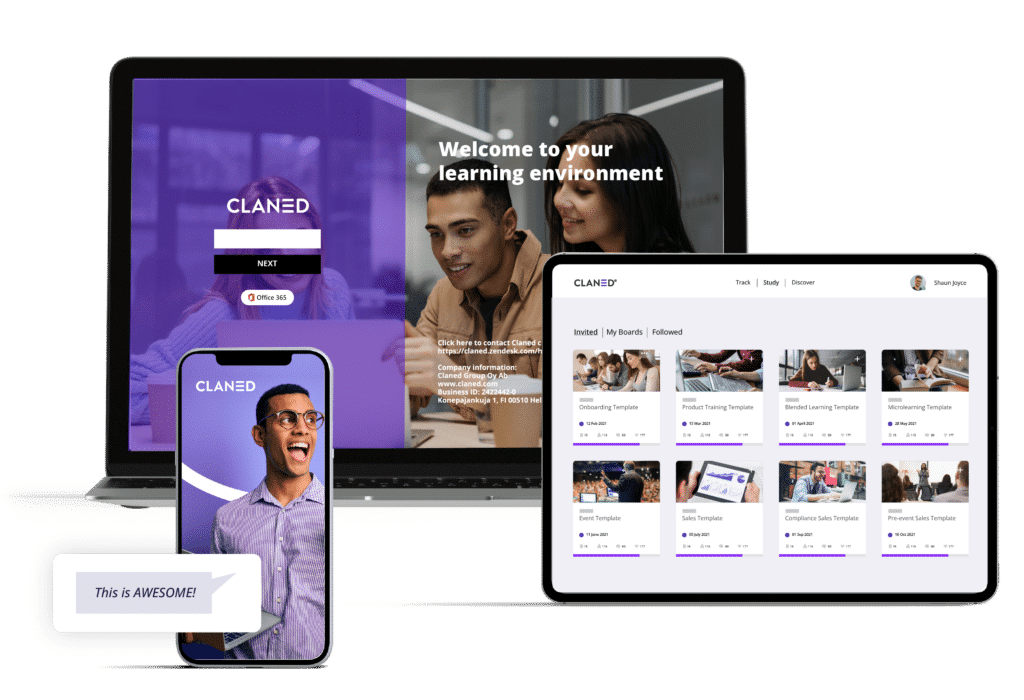
A fresh new look for the front page!
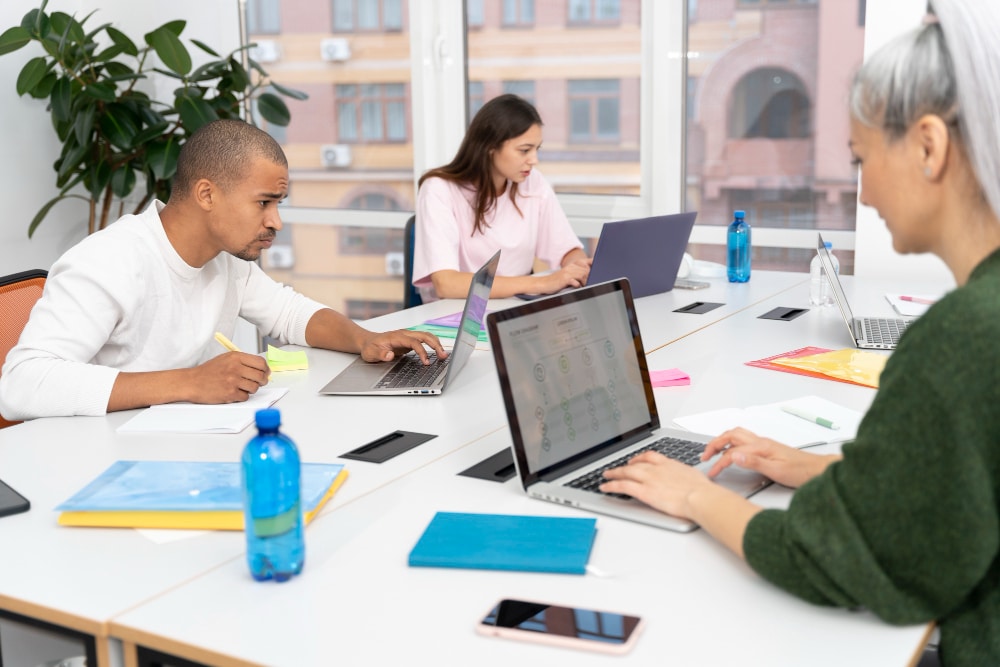

10 Effective Workplace Training Strategies to Boost Employee Performance
Read more news & insights, take a step towards delivering better online learning with claned.
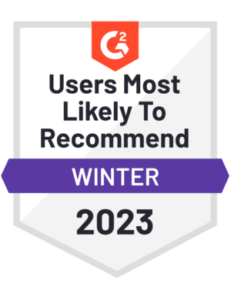
Claned Group Oy Ab Konepajankuja 1 00510 Helsinki sales@claned.com
Business ID: 2422442-0
- Claned Platform
- Learning Design
- Learning Analytics
- Product Training
- HR Training
- Mandatory Training
- Training Companies
- Lessons On Learning
- Learning Clan Podcast
- Claned Academy
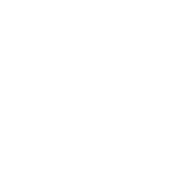
Register Description | Cookies & Personal Data | Privacy Policy | Terms and Conditions
© 2023 Claned. All rights reserved.
Advertisement
Fostering Scientific Literacy and Critical Thinking in Elementary Science Education
- Published: 30 December 2014
- Volume 14 , pages 659–680, ( 2016 )
Cite this article
- Rui Marques Vieira 1 , 2 &
- Celina Tenreiro-Vieira 2
7126 Accesses
63 Citations
3 Altmetric
Explore all metrics
Scientific literacy (SL) and critical thinking (CT) are key components of science education aiming to prepare students to think and to function as responsible citizens in a world increasingly affected by science and technology (S&T). Therefore, students should be given opportunities in their science classes to be engaged in learning experiences that promote SL and CT, which may trigger the need to build and develop knowledge, attitudes/values, thinking abilities, and standards/criteria in an integrated way, resulting in their ability to know how to take responsible action in contexts and situations of personal and social relevance. This paper reports on a study to design, implement, and assess science learning experiences focused on CT toward SL goal. Results support the conclusion that the learning experiences developed and implemented in a grade 6 science classroom had a significant influence on the students’ CT and SL. Within this elementary school context, the theoretical framework used appears to be a relevant and practical aid for developing learning experiences that promote CT/SL and in supporting teaching practices that are more in line with the goals of critical scientific literacy.
This is a preview of subscription content, log in via an institution to check access.
Access this article
Price includes VAT (Russian Federation)
Instant access to the full article PDF.
Rent this article via DeepDyve
Institutional subscriptions
Similar content being viewed by others
Enhancing Scientific Thinking Through the Development of Critical Thinking in Higher Education
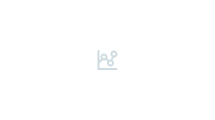
Scientific Thinking and Critical Thinking in Science Education
Developing Higher Order Thinking in Elementary School Science: A Narrative Around a Research Group Quest
Aikenhead, G. (1992). Logical reasoning in science and technology: An academic STS science textbook. Bulletin of Science Technology and Society, 12 (3), 149–159.
Article Google Scholar
Aikenhead, G. (2007, May). Expanding the research agenda for scientific literacy . Paper presented at the Linnaeus Tercentenary Symposium on Promoting Scientific Literacy: Science Education Research in Transaction. Uppsala University, Sweden. Available from http://www.usask.ca/education/profiles/aikenhead/webpage/expand-sl-res-agenda.pdf
American Association for the Advancement of Science (1990). Science for all Americans: Project 2061 . New York, NY: Oxford University Press.
Google Scholar
American Association for the Advancement of Science (1993). Benchmarks for science literacy: Project 2061 . New York, NY: Oxford University Press.
BSCS. (2008). Scientists and science education . Retrieved from http://science.education.nih.gov/SciEdNation.nsf/EducationToday1.html .
Council of Ministers of Education, Canada (1997). Common framework of science learning outcomes, K to 12: Pan-Canadian protocol for collaboration on school curriculum . Retrieved from http://www.cmec.ca/Publications/Lists/Publications/Attachments/177/pancan-protocol-collaboration-1997.pdf
Coutinho, C. (2011). Metodologia de investigação em ciências sociais e humanas [Methodology of research in social sciences and humanities]. Coimbra, Portugal: Almedina.
Department for Education (2011). Review of the National Curriculum in England. What can we learn from English, mathematics and science curricula of high-performing jurisdictions . Retrieved from https://www.gov.uk/government/uploads/system/uploads/attachment_data/file/197636/DFE-RR178a.pdf
Department for Education (2013). National Curriculum to England (framework document) . Retrieved from https://www.gov.uk/government/uploads/system/uploads/attachment_data/file/339805/MASTER_final_national_curriculum_until_sept_2015_11_9_13.pdf
Ministerial Council on Education, Employment, Training and Youth Affairs (2006). National assessment program—Science literacy year 6. School release materials . Author. Retrieved from http://www.scseec.edu.au/archive/Publications/Publications-archive.aspx#measuring
Ennis, R. H. (1987). A taxonomy of critical thinking dispositions and abilities. In J. B. Baron & R. J. Sternberg (Eds.), Teaching thinking skills: Theory and practice . New York, NY: W. H. Freeman.
Ennis, R. H. & Millman, J. (1985). Cornell critical thinking test, level X . Pacific Grove, CA: Midwest.
Finnish National Board of Education (2004). National core curriculum for basic education . Retrieved from http://www.oph.fi/english/sources_of_information/core_curricula_and_qualification_requirements/basic_education
Ford, C. L. & Yore, L. D. (2012). Toward convergence of metacognition, reflection, and critical thinking: Illustrations from natural and social sciences teacher education and classroom practice. In A. Zohar & J. Dori (Eds.), Metacognition in science education: Trends in current research (pp. 251–271). Dordrecht, The Netherlands: Springer.
Chapter Google Scholar
Hackling, M. W., Goodrum, D. & Rennie, L. (2001). The status and quality of teaching and learning of science in Australian schools . Canberra, Australia: Department of Education, Training and Youth Affairs.
Harlen, W. (2006). ASE guide to primary science education . Hatfield, England: Association for Science Education.
Harlen, W. (Ed.). (2010). Principles and big ideas of science education . Hatfield, England: Association for Science Education.
Hatcher, D. & Spencer, L. A. (2000). Reasoning and writing: From critical thinking to composition . Boston, MA: American Press.
Hofstein, A., Eilks, I. & Bybee, R. (2011). Societal issues and their importance for contemporary science education—A pedagogical justification and the state-of-the-art in Israel, Germany, and the USA. International Journal of Science and Mathematics Education, 9 , 1459–1483.
International Council for Science (2011). Report of the ICSU ad-hoc review panel on science. Paris, France: Author. Retrieved from http://www.icsu.org/publications/reports-and-reviews/external-review-of-icsu
Lin, S.-S. (2014). Science and non-science undergraduate students’ critical thinking and argumentation performance in reading a science news report. International Journal of Science and Mathematics Education, 12 , 1023–1046.
Millar, R. & Osborne, J. (1998). Beyond 2000: Science education for the future . London, England: King’s College School of Education.
Ministério da Educação e Ciência (2013). Metas curriculares [Curricular goals]. Lisbon, Portugal: Author.
National Research Council (1996). National science education standards . Washington, DC: National Academies Press.
National Research Council (2012). A framework for K-12 science education: Practices, crosscutting concepts, and core ideas . Washington, DC: National Academies Press.
NGSS Lead States (2013). Next generation science standards: For states, by states . Washington, DC: National Academies Press. Available from http://nextgenscience.org/next-generation-science-standards .
Norris, S. & Ennis, R. H. (1989). Evaluating critical thinking . Pacific Grove, CA: Critical Thinking Press & Software.
Oates, T. (2010). Could do better: Using international comparisons to refine the national curriculum in England . Retrieved from http://www.education.gov.uk/inthenews/inthenews/a0068191/could-do-better-analysis-of-international-curriculums-published .
Organisation for Economic Co-operation and Development (2006a). Assessing scientific, reading and mathematical literacy—A framework for PISA 2006 . Paris, France: Author.
Organisation for Economic Co-operation and Development (2006b). Evolution of student interest in science and technology. Policy report . Paris, France: Retrieved from http://www.oecd.org/dataoecd/16/30/36645825.pdf
Organisation for Economic Co-operation and Development (2009). PISA 2009 assessment framework—Key competencies in reading, mathematics, and science . Paris, France: Author.
Osborne, J. & Dillon, J. (2008). Science education in Europe: Critical reflections . London, England: Nuffield Foundation. Retrieved from http://www.nuffieldfoundation.org/sites/default/files/Sci_Ed_in_Europe_Report_Final.pdf .
Roberts, D. A. (2007). Scientific literacy/science literacy. In S. K. Abell & N. G. Lederman (Eds.), Handbook of research on science education (pp. 729–780). Mahwah, NJ: Lawrence Erlbaum.
Rocard, M., Csermely, P., Jorde, D., Lenzen, D., Walberg-Henriksson, H. & Hemmo, V. (2007). Science education now: A renewed pedagogy for the future of Europe . Luxembourg, Belgium: European Commission. Retrieved from http://ec.europa.eu/research/science-society/document_library/pdf_06/report-rocard-on-science-education_en.pdf .
Scriven, M. & Paul, R. (2007). Defining critical thinking . Retrieved from http://www.criticalthinking.org/aboutCT/define_critical_thinking.cfm .
Tenreiro-Vieira, C. & Vieira, R. M. (2011). Educação em ciências e em matemática numa perspectiva de literacia: desenvolvimento de materiais didácticos CTS / Pensamento Crítico (PC) [Mathematics and science education for literacy]. In W. dos Santos & D. Auler (Eds.), CTS e educação científica: desafios, tendências e resultados de pesquisas (pp. 417–437). Brasília, Brazil: Editora Universidade de Brasília.
Vieira, R. M. (1995). O desenvolvimento de courseware promotor de capacidades de pensamento crítico [The development of a courseware to promote critical thinking abilities] (Unpublished master’s thesis). University of Lisbon, Portugal .
Vieira, R. M., Tenreiro-Vieira, C. & Martins, I. (2011). Educação em ciências com orientação CTS [Science education with STS orientation]. Porto, Portugal: Areal Editores.
Yore, L. D. (2012). Science literacy for all—More than a slogan, logo, or rally flag! In K. C. D. Tan & M. Kim (Eds.), Issues and challenges in science education research: Moving forward (pp. 5–23). Dordrecht, The Netherlands: Springer.
Yore, L. D., Pimm, D. & Tuan, H.-L. (2007). The literacy component of mathematical and scientific literacy. International Journal of Science and Mathematics Education, 5 , 559–589.
Download references
Acknowledgments
The authors thank Dr. Larry Yore and Mrs. Sharyl Yore for their mentoring assistance.
Author information
Authors and affiliations.
Department of Education, University of Aveiro, 3810-193, Aveiro, Portugal
Rui Marques Vieira
Research Centre for Didactics and Technology in Teacher Education, University of Aveiro, Aveiro, Portugal
Rui Marques Vieira & Celina Tenreiro-Vieira
You can also search for this author in PubMed Google Scholar
Corresponding author
Correspondence to Rui Marques Vieira .
Rights and permissions
Reprints and permissions
About this article
Vieira, R.M., Tenreiro-Vieira, C. Fostering Scientific Literacy and Critical Thinking in Elementary Science Education. Int J of Sci and Math Educ 14 , 659–680 (2016). https://doi.org/10.1007/s10763-014-9605-2
Download citation
Received : 30 April 2014
Accepted : 13 November 2014
Published : 30 December 2014
Issue Date : May 2016
DOI : https://doi.org/10.1007/s10763-014-9605-2
Share this article
Anyone you share the following link with will be able to read this content:
Sorry, a shareable link is not currently available for this article.
Provided by the Springer Nature SharedIt content-sharing initiative
- Critical thinking
- Elementary science education
- Scientific literacy
- Find a journal
- Publish with us
- Track your research
What’s the Difference Between Critical Thinking and Scientific Thinking?
Thinking deeply about things is a defining feature of what it means to be human, but, surprising as it may seem, there isn’t just one way to ‘think’ about something; instead, humans have been developing organized and varied schools of thought for thousands of years.
Discussions about morality, religion, and the meaning of life often drive knowledge-seeking inquiry, leading people to wonder what the difference is between critical thinking and Scientific Thinking.
Critical thinkers prioritize objectivity to analyze a problem, deduce logical solutions, and examine what the ramifications of those solutions are.
While scientific thinking often relies heavily on critical thinking, scientific inquiry is more dedicated to acquiring knowledge rather than mere abstraction.
What Is Critical Thinking?
A critical thinker may discern what they already know about a subject, what that information suggests, why that information is relevant, and how that information could be linked to further lines of inquiry. Critical thinking is, therefore, simply the ability to think clearly and logically.
Naturally, the ability to think critically is highly prized in an academic setting, and most educators seek to enable their students to think critically.
So much information can be interlinked to develop our understanding of the world, and critical thinking is the basis for using objectivity to not only establish likely outcomes to a scenario, but also inquire on the repercussions of that outcome and reflect on the process by which one came to that conclusion.
What Is Scientific Thinking?
As you might imagine, this process can be repeated ad infinitum. So, you draw a conclusion that’s scientifically verifiable? Great! Now you can take that conclusion and use it as a basis for a new experiment. Of course, the scientific method has limits.
Physics is known as the perfect science because the forces that comprise our world are well understood and don’t tend to exhibit anomalies, making the empirically verified scientific method perfect for improving our understanding of the natural world.
How Are Critical Thinking and Scientific Thinking Similar and Different?
The main difference between the two, however, is the goal of each discipline.
Both scientific thinking and critical thinking tend to draw links between concepts, evaluating how they are related and what knowledge may be gleaned from that connection.
Scientific thinkers develop a hypothesis, test it, and then rinse and repeat until the phenomenon is understood. As such, scientific thinkers are obsessed with why questions. Why does this phenomenon happen?
Why does matter behave like this? In the end, both schools are thought have a lot of interesting ideas guiding them, and most of us probably use them throughout our daily lives.
https://psycnet.apa.org/record/2010-22950-019
You may also like
The relationship between empathy and critical thinking: a balanced approach, thinking critically about your personal finance in a recession, tips on how to use critical thinking in reading and writing, 10 critical thinking questions you can use to solve everyday problems, download this free ebook.

In order to continue enjoying our site, we ask that you confirm your identity as a human. Thank you very much for your cooperation.
Get the Reddit app
Critical Thinking 911
HOW CRITICAL THINKING FACILITATES THE ACQUISITION OF SCIENTIFIC KNOWLEDGE?
Introduction
Critical thinking is a cognitive process that involves analyzing, evaluating, and synthesizing information to form judgments and make decisions. It is an essential component of scientific inquiry, as it allows individuals to evaluate the credibility of scientific claims and evidence. In this article, we will discuss how critical thinking facilitates the acquisition of scientific knowledge.
Critical Thinking and Scientific Method
The scientific method is a systematic approach to acquiring knowledge that involves formulating a hypothesis, testing it through experiments, analyzing the results, and drawing conclusions. Critical thinking is an integral part of this process, as it allows individuals to evaluate the validity and reliability of scientific claims and evidence. The ability to think critically enables individuals to assess the quality of evidence and to identify any potential biases or errors in scientific research.
For example, a critical thinker might question the methods used in a scientific study, such as the sample size or the control group. They might also examine the validity of the data presented, such as statistical significance or the reproducibility of the results. By engaging in this type of critical analysis, individuals can make more informed decisions about the credibility of scientific claims.
Critical Thinking and Skepticism
Skepticism is another important component of critical thinking in the context of scientific inquiry. Skepticism involves questioning assumptions, challenging beliefs, and demanding evidence to support claims. It is an essential tool for scientific inquiry, as it allows individuals to separate fact from fiction and to avoid being misled by false or misleading information.
For example, a critical thinker might be skeptical of claims made by alternative medicine practitioners or conspiracy theorists. They might demand evidence to support such claims and evaluate the credibility of the sources cited. By engaging in this type of skeptical analysis, individuals can avoid being misled by unsupported or false claims.
Critical Thinking and Logical Reasoning
Logical reasoning is another key component of critical thinking in the context of scientific inquiry. Logical reasoning involves using deductive or inductive reasoning to evaluate the validity of arguments or claims. It is an essential tool for scientific inquiry, as it allows individuals to identify flaws or weaknesses in arguments and to evaluate the strength of evidence.
For example, a critical thinker might use logical reasoning to evaluate an argument presented in a scientific study. They might examine the premises and conclusions of the argument, identify any flaws or weaknesses, and evaluate the strength of the evidence presented. By engaging in this type of logical analysis, individuals can make more informed decisions about the validity of scientific claims.
Critical Thinking and Cognitive Biases
Cognitive biases are another important consideration in the context of critical thinking in scientific inquiry. Cognitive biases are tendencies to think in certain ways that can lead to errors in judgment and decision-making. They can affect the way individuals interpret and evaluate scientific information, leading to misconceptions or false beliefs.
For example, confirmation bias is a cognitive bias that involves seeking out information that confirms pre-existing beliefs while ignoring information that contradicts them. This bias can lead individuals to accept scientific claims that support their pre-existing beliefs while rejecting claims that contradict them. By being aware of cognitive biases and engaging in critical thinking, individuals can avoid being misled by these biases and make more informed decisions about scientific claims.
Critical thinking is an essential component of scientific inquiry that facilitates the acquisition of scientific knowledge. By engaging in critical analysis, skepticism, logical reasoning, and by being aware of cognitive biases, individuals can evaluate the credibility of scientific claims and evidence. This allows individuals to make more informed decisions about scientific claims, and to avoid being misled by false or misleading information.
Have no time to work on your critical thinking? Well, we do. We will write an critical thinking sample crafted to your needs. In-time submission and academic quality guaranteed. - EditaPaper.com
Academia.edu no longer supports Internet Explorer.
To browse Academia.edu and the wider internet faster and more securely, please take a few seconds to upgrade your browser .
Enter the email address you signed up with and we'll email you a reset link.
- We're Hiring!
- Help Center
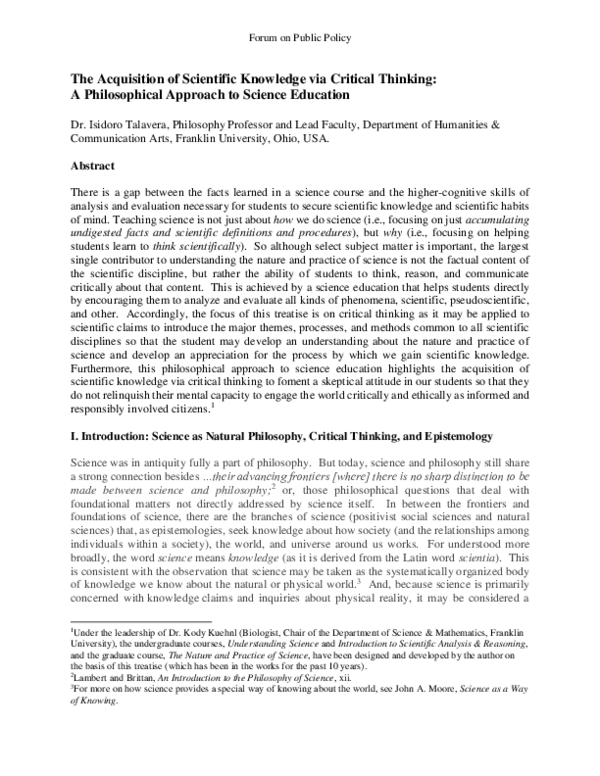
The Acquisition of Scientific Knowledge Via Critical Thinking: A Philosophical Approach to Science Education

2016, Forum on Public Policy: A Journal of the Oxford Round Table
Related Papers
Thelma Gunn
Many North American school districts and post-secondary academic institutions are acknowledging the importance of becoming a critical thinker. Future citizens will need to be to informed consumers of technology, science, sociology, and ethics, to name a few. After all, the world has become vastly more complicated, necessitating such skills as reasonableness and logical thinking. By engaging students at a crucial time in their developmental process, we can lay the foundation for good critical thinkers. The purpose of this paper is to examine the importance of critical thinking in science education, both at the secondary and post-secondary levels. Evidence regarding its suitability will be drawn from critical thinking and science education literature, as well as previous studies using bioethical decision-making and generic question stem strategies with middle school and university students.
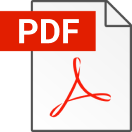
In different countries efforts have been made to integrate critical thinking into science curricula, recognizing that it is necessary to live in a plural society with citizenship competence. However, this objective has not been appropriately implemented in classrooms. One of the obstacles is the fact that teachers do not have a clear idea about critical thinking because the meaning ascribed to critical thinking in different contexts is rarely explicit. This paper attempts to clarify this concept, evincing its relationship with other concepts such as scientific literacy and to present and discuss a framework for promoting students’ critical thinking in science classrooms.
lance grigg
Peter Ellerton
Redefining Scientific Thinking for Higher Education
Kerry-Ann R Douglas-Lindsay
Revolutionary thinkers must never cease to question as the great philosopher and thinker Socrates once said, “Wisdom begins in wonder”. In this technologically information saturated world it is quite easy to become complacent and lax in our thinking, accepting everything presented to us, however, it is foolish for anyone to be so gullible even if the source is considered reputable. As educators we must inculcate in the minds of our students a mentality which fosters wonderment, inquiry and a drive to finding answers to questions. The sciences are a perfect course to achieve this in the young; through inquiry and questioning the minds of the young can be molded and shaped into great thinkers such as Socrates who was not fearful of the dominant culture and was courageous in these pursuits to question the hegemonic forces of the day. This study seeks to find out strategies that teachers can employ to promote critical thinking in students. Studies have shown that this skill is seriously lacking in both the young and old and is therefore limiting the potential of those individuals in achieving their fullest potential. Critical thinking is a natural thing in some but it is also a learnt skill as well coupled with these two known facts is the notion that no one will always get it right, but with this skill one can prepare themselves for uncertainty and minimize the negative effects of an uncritical mind. The mind of a critical thinker is one that is aware of the fact that they, no matter how much they think they know knows very little. The title of this Action Research is Developing Critical Thinking in a Group of 11 Grade Science Students using the Inquiry Based Science Education (IBSE) approach. This study will utilize a mixed method research design (both qualitative and quantitative approaches). The population for this study will come from two of my grade 11 science classes each consisting of approximately 30 students. The average age of the students in both classes is 16 years. The reason for this study is to find out whether the IBSE approach can develop students critical thinking. It is hoped that the findings of the study will benefit my practice and my students both present and future. The benefits I hope to achieve include: improvement in my students critical thinking, improvements in my instructional delivery and improvement in the academic performance of my students. Over the past ten years I have observed poor critical thinking amongst students, particularly in their analysis and interpretation skill component in the Caribbean Secondary Examination Council (CSEC) School Based Assessment (SBA), and in their application of scientific knowledge. These two areas are necessary components of how to think critically, especially when answering higher order thinking questions; individuals must be able to analyze, synthesize, interpret, solve problems, and evaluate concepts and issues.
CBE life sciences education
Steven Koether
Recent studies question the effectiveness of a traditional university curriculum in helping students improve their critical thinking and scientific literacy. We developed an introductory, general education (gen ed) science course to overcome both deficiencies. The course, titled Foundations of Science, differs from most gen ed science offerings in that it is interdisciplinary; emphasizes the nature of science along with, rather than primarily, the findings of science; incorporates case studies, such as the vaccine-autism controversy; teaches the basics of argumentation and logical fallacies; contrasts science with pseudoscience; and addresses psychological factors that might otherwise lead students to reject scientific ideas they find uncomfortable. Using a pretest versus posttest design, we show that students who completed the experimental course significantly improved their critical-thinking skills and were more willing to engage scientific theories the general public finds contro...
Science Education
Dana Zeidler
Robert Swartz
Interdisciplinary Journal of Multidisciplinary Research and Publications
Mc Rollyn Vallespin
The significance of fostering critical thinking in scientific education cannot be overstated in today's rapidly evolving technological landscape. The researcher conducts a comprehensive bibliometric analysis, scrutinizing 220 scholarly articles, to deepen the understanding of research in critical thinking within science education. This analysis reveals the foremost authors, influential journals, and pivotal keywords, offering insights into the primary contributors and central themes in this field. Furthermore, the global distribution of research on critical thinking in science education is explored, shedding light on the substantial and diverse contributions from various regions and academic institutions. The findings emphasize the need for an increased focus on nurturing critical thinking skills and their practical applications within educational contexts. To enhance science education, a multifaceted approach is proposed. This includes the strategic integration of technology into curricula, the exploration of innovative pedagogical methods such as the Flipped Classroom model, the incorporation of constructed- response questions in assessment techniques, and the facilitation of global collaboration among researchers, educators, and policymakers. The study goes beyond these recommendations to advocate for a more holistic student development approach. Interdisciplinary collaboration is encouraged, and a student-centered approach to teaching and learning is endorsed. Following these recommendations aims to enrich science education and empower students and educators to navigate the complexities of the contemporary scientific landscape effectively.
Loading Preview
Sorry, preview is currently unavailable. You can download the paper by clicking the button above.
RELATED PAPERS
Jonte Taylor
Science & Education
Peter Logan , Mal Hooper
Calvin Kalman
Tuti Hardianti
Journal of Science Education and Technology
David Ben-Chaim
Rosemary Hipkins
meeting of the National …
Mary Anne Rea-Ramirez
Tom Murray , Matthew Mattingly
ProTiM PPD Kudat
Gábor Zemplén
Electronic Journal of Research in Educational Psychology,
Acta Didactica Napocensia
Natassa Raikou , Konstantinos Ravanis
Cultural Studies of Science Education
Hagop Yacoubian
J. Turk. Sci. Edu
Mustafa Cakir
Journal of Development and Social Sciences
Naima Qureshi
RELATED TOPICS
- We're Hiring!
- Help Center
- Find new research papers in:
- Health Sciences
- Earth Sciences
- Cognitive Science
- Mathematics
- Computer Science
- Academia ©2024

IMAGES
VIDEO
COMMENTS
The Problem of Induction is a problem for science because [s]cientific methodology generally starts from the observation of particular events and arrives at a generalization by the process of induction.28 In view of that, the Problem of Induction may be expressed in the following form. 1. All observed F's are G's.
Developing critical-thinking and scientific reasoning skills are core learning objectives of science education, but little empirical evidence exists regarding the interrelationships between these constructs. Writing effectively fosters students' development of these constructs, and it offers a unique window into studying how they relate.
There is a gap between the facts learned in a science course and the higher-cognitive skills of analysis and evaluation necessary for students to secure scientific knowledge and scientific habits of mind. Teaching science is not just about how we do science (i.e., focusing on just "accumulating undigested facts and scientific definitions and procedures"), but "why" (i.e., focusing on helping ...
Scientific thinking and critical thinking are two intellectual processes that are considered keys in the basic and comprehensive education of citizens. For this reason, their development is also contemplated as among the main objectives of science education. However, in the literature about the two types of thinking in the context of science education, there are quite frequent allusions to one ...
Science as Natural Philosophy, Critical Thinking, and Epistemology Science was in antiquity fully a part of philosophy, but today, science and philosophy still share a strong connection besides ... their advancing frontiers. I. Introduction: Science as Natural Philosophy, Critical Thinking, and Epistemology Science was in antiquity fully a part of philosophy. But today, science and philosophy ...
History. This supplement elaborates on the history of the articulation, promotion and adoption of critical thinking as an educational goal. John Dewey (1910: 74, 82) introduced the term 'critical thinking' as the name of an educational goal, which he identified with a scientific attitude of mind. More commonly, he called the goal ...
studies, authors advocate adopting critical thinking as the course framework (Pukkila, 2004) and developing explicit examples of how critical thinking relates to the scientific method (Miri et al., 2007). In these examples, the important connection between writ-ing and critical thinking is highlighted by the fact that each
Abstract. This paper reviews some of the most critical issues in science in terms of scientific thinking, and. reasoning. Many students arrive at college poorly prepared to function in the typical ...
traditional rule provides us with a critical way to iden-tify either ignorance of the scientific background of the work or the presence in the text of hidden agendas that have nothing to do with science. In practice, the initiation of the process of critical thinking ought to begin with a step similar to the 'due dil -
They write: 'the primary goal of training in critical thinking is an epistemic transformation that is not merely the acquisition of new knowledge, but the adoption of a new and better way of reasoning' (Paul and Quiggin, 2020: 572). By developing critical thinking skills, students develop the reasoning tools that can reorient their beliefs ...
Research publications, policy papers and reports have argued that higher education cannot only facilitate learning of domain-specific knowledge and skills, but it also has to promote learning of thinking skills for using that knowledge in action (e.g. Greiff et al., 2014; Shavelson, 2010a; Strijbos, Engels, & Struyven, 2015).The focus on critical thinking arises, in part, because of higher ...
The discrepancies This 1 Why (NOS) in school science Findings Role assertion According is of important Critical deemed is to based Hag Thinking Critical crucial on p some A. Yacoubian thinking linked and in authors the to increasingly context in the context Critical affirmations, (2015), of Science, thinking. are present there of as Science ...
Evaluation: Critical thinkers assess the credibility and logical strength of evidence, arguments, and claims. They discern bias, identify inconsistencies, and evaluate the validity of sources and content. Inference: The ability to draw reasoned conclusions from the information at hand is a crucial aspect of critical thinking.
Abstract. Scientific literacy (SL) and critical thinking (CT) are key components of science education aiming to prepare students to think and to function as responsible citizens in a world increasingly affected by science and technology (S&T). Therefore, students should be given opportunities in their science classes to be engaged in learning ...
Critical thinkers prioritize objectivity to analyze a problem, deduce logical solutions, and examine what the ramifications of those solutions are. While scientific thinking often relies heavily on critical thinking, scientific inquiry is more dedicated to acquiring knowledge rather than mere abstraction. There are a lot of nuances between ...
control of knowledge acquisition, and speed of knowledge acquisition. According to Hofer and Pintrich (1997), the last two dimensions presented by Schommer-Aikins are directly related to learning and cannot be included in the scope of personal epistemology. ... The relationship between critical thinking, scientific epistemological beliefs, and the
One of the essential components of science literacy is the ability to think and evaluate "critically" scientific claim and evidence. To think critically requires deliberative and controlled cognitive process which needs much cognitive efforts people often rely on heuristic (sometimes biased) processing, so that previous investigations showed that people were susceptible to pseudoscientific ...
Accordingly, the focus of this treatise is on critical thinking as it may be applied to scientific claims to introduce the major themes, processes, and methods common to all scientific disciplines so that the student may develop an understanding about the nature and practice of science and develop an appreciation for the process by which we ...
Answer and Explanation: 1. Become a Study.com member to unlock this answer! Create your account. View this answer. Critical thinking involves the analysis of an idea based on the facts gathered about that idea. Scientific knowledge is knowledge obtained using the... See full answer below.
Introduction Critical thinking is a cognitive process that involves analyzing, evaluating, and synthesizing information to form judgments and make…
Authors like Davson-Galle (2004) and Marples (1999), write that for many years, the objectives in education were supported by liberalism and centered on autonomy. Currently, according to the last author, these objectives have expanded and include: social justice, national identity, the curriculum, social practices and critical thinking.
Over the past ten years I have observed poor critical thinking amongst students, particularly in their analysis and interpretation skill component in the Caribbean Secondary Examination Council (CSEC) School Based Assessment (SBA), and in their application of scientific knowledge.
First, define critical thinking. Discuss how critical thinking facilitates the acquisition of scientific knowledge.. Use the four critical questions to analyze and evaluate if the claims you chose are justified (refer to the examples in the textbook and key points to see how to analyze and evaluate claims using the four critical questions).Lack ...