Impacts and Responses of Volcanic Activity ( AQA A Level Geography )
Revision note.
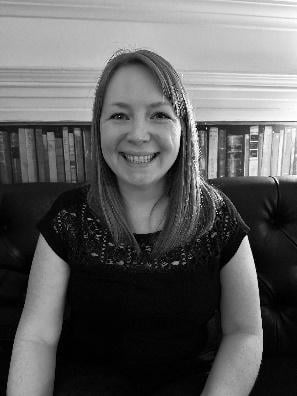
Geography Content Creator

Primary & Secondary Impacts of Eruptions
- Primary impacts are the immediate effects of the hazard such as injuries and deaths, and damage to property and infrastructure
- Secondary impacts are the longer-term effects of the hazard such as economic losses, social and psychological consequences
Examples of Impacts of Volcanic Hazards
|
|
|
| Deaths and injuries Displacement of people Damage to properties and infrastructure causing disruption to people’s lives
| Mental health issues e.g. stress, anxiety, depression Homelessness Disruption to services such as healthcare and education Loss of cultural heritage sites |
| Destruction of property and infrastructure leading to significant costs for repair and reconstruction Disruption of trade and economic activity Loss of income and employment Costs of immediate responses e.g. food aid, temporary shelter, medical treatment | Slower economic growth and development Increased economic inequality as impacts are more severe for most vulnerable people Increased costs of insurance and hazard management strategies |
| Poor air and water quality Loss of biodiversity Destruction of habitats and ecosystems
| Acid rain can damage ecosystems Release of greenhouse gases can lead to a rise in global temperatures Ash clouds can lead to a fall in global temperatures due to solar energy being reflected back into space |
| Pressure on governments to co-ordinate emergency response Social unrest and political instability | Conflicts over government response and food shortage Changes in government policy and regulations to reduce future risk |
Case Study - Mount Merapi, Indonesia
- Name - Mount Merapi
- Location - Java, Indonesia
- Date - 25th October -30th November 2010
- Magnitude - VEI 4
- Plate boundary - Destructive plate boundary where the Indo-Australian plate is subducting below the Eurasian plate
- Type of volcano - Stratovolcano or composite
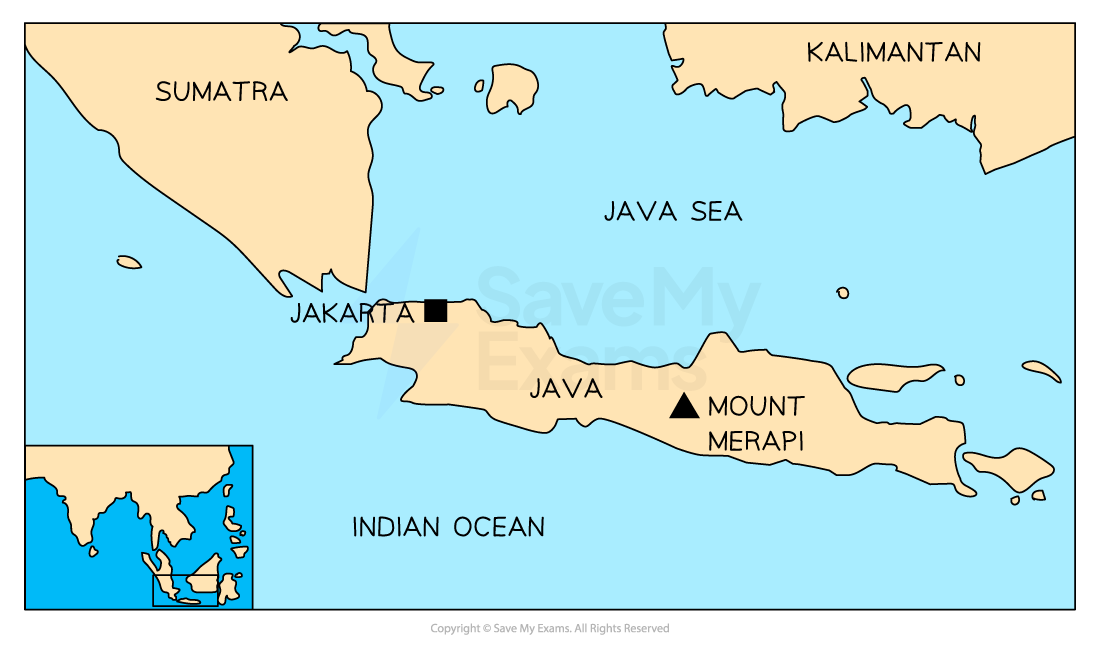
Location of Mount Merapi
Impacts of the 2010 Eruption of Mount Merapi, Indonesia
|
|
|
| sulphur dioxide gas caused skin irritation and breathing problems | |
| ||
| ||
|
Long & Short-Term Responses to Volcanic Hazards
- Long-term responses to volcanic hazards are measures taken to reduce the impacts of future eruptions
- Short-term responses usually happen immediately before, during or after a volcanic eruption
Examples of Long and Short-term Responses
|
|
– identifying areas at most risk and limiting the activities that can take place there – creating a set of building codes to increase the resilience of buildings to volcanic hazards – preparing communities to improve evacuation and response | – moving people out of the danger zone around a volcano when it shows signs of erupting – using sniffer dogs and heat sensors to locate survivors and rescue them - providing food, water, medical aid to affected communities
|
- Responses can also be categorised as prevention, preparedness, mitigation and adaptation
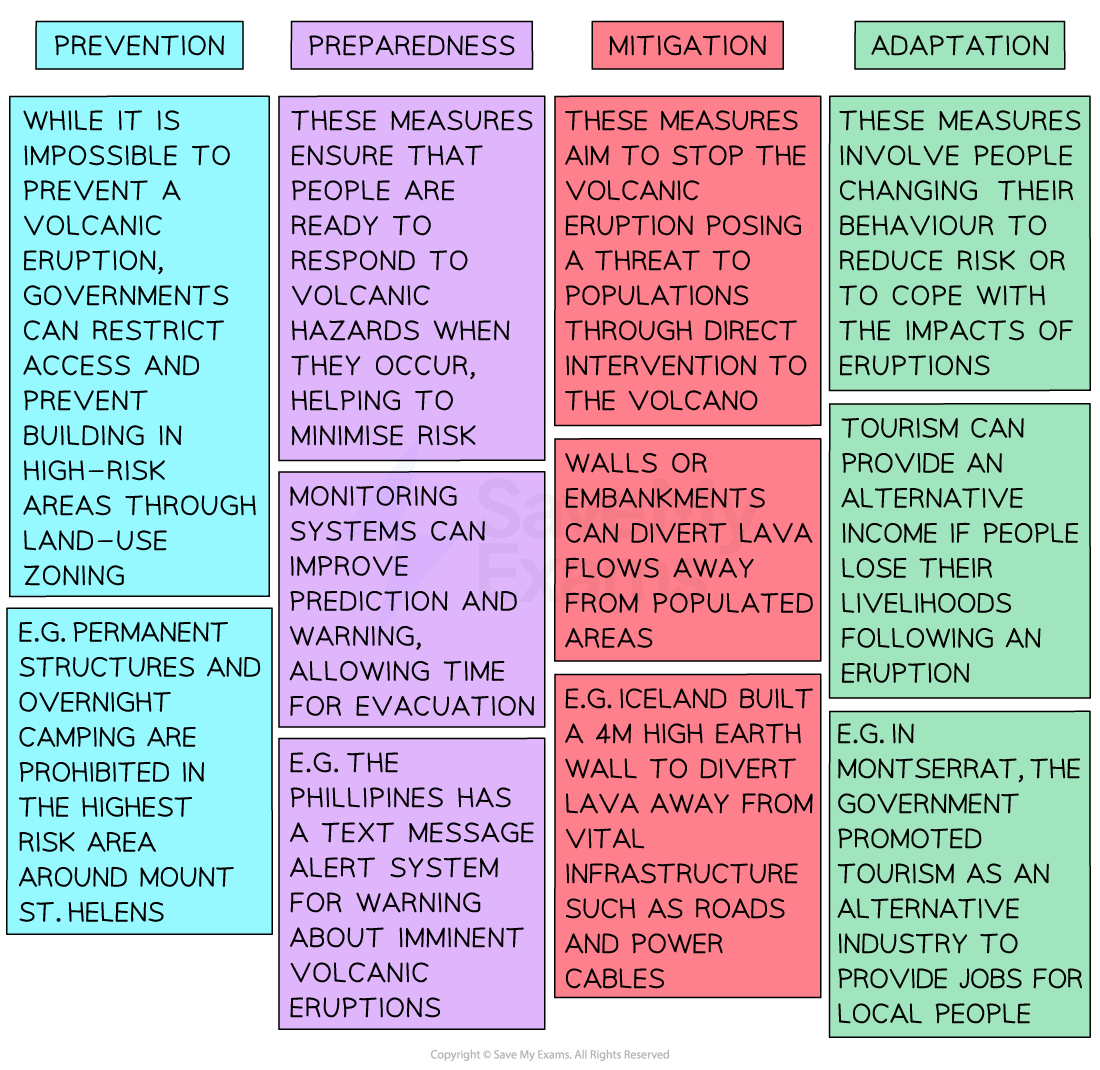
Responses to volcanic hazards
- Short-term responses included:
- Evacuation of over 350,000 people
- Over 200 temporary shelters were set up to provide shelter to displaced people
- Police and military were mobilised to provide emergency assistance such as food, shelter and medical care
- Aid agencies such as the Indonesian Red Cross distributed emergency aid e.g. blankets and food
- Long-term responses included:
- Financial assistance was provided to help people rebuild their homes
- 16,000 new homes were built by the government to replace those lost
- Damaged infrastructure such as roads and schools were repaired or replaced
- A new volcano monitoring centre was established to improve prediction and warnings
- An early warning system was created to alert people in high-risk areas
- Evacuation plans were improved with signposted routes and shelter locations
- Marketing campaigns were used to promote tourism and aid economic recovery
You've read 0 of your 0 free revision notes
Get unlimited access.
to absolutely everything:
- Downloadable PDFs
- Unlimited Revision Notes
- Topic Questions
- Past Papers
- Model Answers
- Videos (Maths and Science)
Join the 100,000 + Students that ❤️ Save My Exams
the (exam) results speak for themselves:
Did this page help you?
Author: Rhiannon Molyneux
Rhiannon graduated from Oxford University with a BA in Geography before training as a teacher. She is enthusiastic about her subject and enjoys supporting students to reach their full potential. She has now been teaching for over 15 years, more recently specialising at A level. Rhiannon has many years of experience working as an examiner for GCSE, IGCSE and A level Geography, so she knows how to help students achieve exam success.
Volcano case studies
Volcano case studies You should make sure you are familiar with 2 case studies: Either: Nyiragongo, Democratic Republic of Congo – Poor Country or Montserrat, Caribbean – Poor Country AND Either: Mount St. Helens, USA – Rich Country or Iceland – Rich Country
Key terms: Primary effects: the immediate effects of the eruption, caused directly by it Secondary effects: the after-effects that occur as an indirect effect of the eruption on a longer timescale Immediate responses: how people react as the disaster happens and in the immediate aftermath Long-term responses: later reactions that occur in the weeks, months and years after the event Nyiragongo Picture The video below contains more information on the primary and secondary effects of a volcano
On 17th January 2002 Nyiragongo volcano in the Democratic Republic of Congo (DRC) was disturbed by the movement of plates along the East African Rift Valley. This led to lava spilling southwards in three streams.
The primary effects – The speed of the lava reached 60kph which is especially fast. The lava flowed across the runway at Goma airport and through the town splitting it in half. The lava destroyed many homes as well as roads and water pipes, set off explosions in fuel stores and powerplants and killed 45 people
The secondary effects – Half a million people fled from Goma into neighbouring Rwanda to escape the lava. They spent the nights sleeping on the streets of Gisenyi. Here, there was no shelter, electricity or clean water as the area could not cope with the influx. Diseases such as cholera were a real risk. People were frightened of going back. However, looting was a problem in Goma and many residents returned within a week in hope of receiving aid.
Responses – In the aftermath of the eruption, water had to be supplied in tankers. Aid agencies, including Christian Aid and Oxfam, were involved in the distribution of food, medicine and blankets.
Montserrat – Poor country case study
Montserrat – Ledc Case Study from donotreply16 Mount St Helens – Rich country case study Picture Mount St. Helens is one of five volcanoes in the Cascade Range in Washington State, USA. The volcano erupted at 8:32am on 18th May 1980.
Effects – An earthquake caused the biggest landslide ever recorded and the sideways blast of pulverised rock, glacier ice and ash wiped out all living things up to 27km north of the volcano. Trees were uprooted and 57 people died.
Immediate responses – helicopters were mobilised to search and rescue those in the vicinity of the catastrophic blast. Rescuing survivors was a priority, followed by emergency treatment in nearby towns. Air conditioning systems were cleaned after by clogged with ash and blocked roads were cleared. Two million masks were ordered to protect peoples lungs.
Long-term responses – Buildings and bridges were rebuilt. Drains had to be cleared to prevent flooding. The forest which was damaged had to be replanted by the forest service. Roads were rebuilt to allow tourists to visit. Mount St. Helens is now a major tourist attraction with many visitor centres.
Iceland – Rich country case study Picture Location: Iceland lies on the Mid-Atlantic Ridge, a constructive plate margin separating the Eurasian plate from the North American plate. As the plates move apart magma rises to the surface to form several active volcanoes located in a belt running roughly SW-NE through the centre of Iceland. Eyjafjallajokull (1,666m high) is located beneath an ice cap in southern Iceland 125km south east of the capital Reykjavik
The Eruption: In March 2010, magma broke through the crust beneath Eyjafjallajokull glacier. This was the start of two months of dramatic and powerful eruptions that would have an impact on people across the globe. The eruptions in March were mostly lava eruptions. Whilst they were spectacular and fiery they represented very little threat to local communities, However, on 14th April a new phase began which was much more explosive. Over a period of several days in mid-April violent eruptions belched huge quantities of ash in the atmosphere.
Local impacts and responses: The heavier particles of ash (such as black gritty sand) fell to the ground close to the volcano, forcing hundreds of people to be evacuated (immediate response) from their farms and villages. As day turned to night, rescuers wore face masks to prevent them choking on the dense cloud of ash. These ash falls, which coated agricultural land with a thick layer of ash, were the main primary effects of the eruption. One of the most damaging secondary effects of the eruption was flooding. As the eruption occurred beneath a glacier, a huge amount of meltwater was produced. Vast torrents of water flowed out from under the ice. Sections of embankment that supported the main highway in Southern Iceland were deliberately breached by the authorities to allow floodwaters to pass through to the sea. This action successfully prevented expensive bridges being destroyed. After the eruption, bulldozers were quickly able to rebuild the embankments and within a few weeks the highway was reconstructed.
Local impacts: 800 people evacuated Homes and roads were damaged and services (electricity & water) disrupted Local flood defences had to be constructed Crops were damaged by heavy falls of ash Local water supplies were contaminated with fluoride from the ash
National impacts: Drop in tourist numbers – affected Iceland’s economy as well as local people’s jobs and incomes Road transport was disrupted as roads were washed away by floods Agricultural production was affected as crops were smothered by a thick layer of ash Reconstruction of roads and services was expensive
International impacts: Over 8 days – some 100,000 flights were cancelled 10 million air passengers affected Losses estimated to be £80 million Industrial production halted due to a lack of raw materials Fresh food could not be imported Sporting events such as the Japanese Motorcycle grand prix, Rugby leagues challenge cup and the Boston Marathon were affected
International impacts and responses: The eruption of Eyjafjallajokull became an international event in mid-April 2010 as the cloud of fine ash spread south-eastwards toward the rest of Europe. Concerned about the possible harmful effects of ash on aeroplane jet engines, large sections of European airspace closed down. Passenger and freight traffic throughout much of Europe ground to a halt. The knock-on effects were extensive and were felt across the world. Business people and tourists were stranded unable to travel in to or out of Western Europe. Industrial production was affected as raw materials could be flown in and products could not be exported by air. As far away as Kenya, farm workers lost their jobs or suffered pay cuts as fresh produce such as flowers and bean perished, unable to be flown to European supermarkets. The airline companies and airport operators lost huge amounts of money. Some people felt that the closures were an over-reaction and that aeroplanes could fly safely through low concentrations of ash. However, a scientific review conducted after the eruption concluded that under the circumstances it had been right to close the airspace. Further research will be carried out as a long-term response to find better ways of monitoring ash concentrations and improving forecast methods.
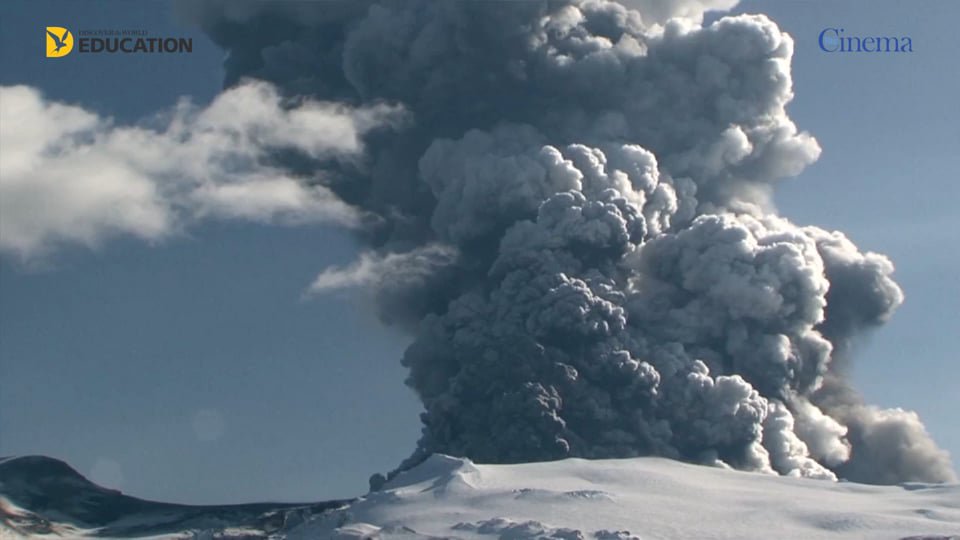
Eyjafjallajokull 2010 volcanic eruption case study
Tectonic Hazards Community Insight
Provided in partnership with
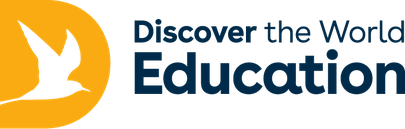
- For partners
- For partner schools (coming soon)
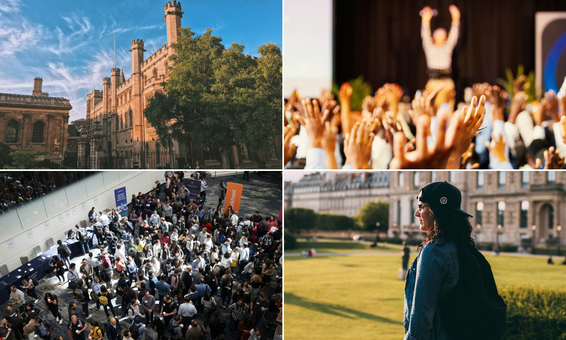
Geography Events Calendar
Upcoming open days, lectures and careers events
Log in or sign up to manage your videos and for new video alerts Log in Sign up
Course links: GCSE • IGCSE • A-level • IA-level • IB Geography
This award-winning geography case study video resource reflects on the eruption of Eyjafjallajokull in 2010 and looks ahead to potential volcanic eruptions in Iceland.
In this video, we cover:
- The causes and impacts of the eruption, with visits to some of the localities directly affected - Volcano monitoring and preparedness - The impacts associated with the future eruption of Katla - Positive impacts of the volcanic eruption on tourism in Iceland
This teaching resource uses narrative, incisive interviews of local people, stunning archive footage of the eruption itself and supportive maps and diagrams to show that, through detailed scientific knowledge and monitoring, the people in Iceland not only understand the threats posed by volcanic eruptions but also see the rich benefits of living in the ‘Land of Fire and Ice’.
Visit Discover the World Education to download the free teaching resources, which accompany this video: http://bit.ly/2xzJ8r5
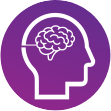
Personalised lessons and regular feedback to ensure you ace your exams! Book a free consultation today
Gain hands-on experience of how subjects are used in different fields. Experience life as a uni student and boost your university application with our summer programme!
- Revision notes >
- IGCSE Geography Revision Notes >
- Theme 2: Natural Environment
VOLCANO case study: Mt Soufriere, Montserrat 1997
Causes of eruption .
The island has been created because the Caribbean Plate and Atlantic Plate are moving towards each other and the dense oceanic plate is being subducted under the lighter continental plate.
At destructive boundaries oceanic crust is destroyed as it is forced below the less dense continental crust. The partially melted rock forces its way to an area of lower pressure ready to erupt.
Before 1995 Mount Soufriere had been dormant for over 300 years.
In 1995 the volcano began to give off warning signs of an eruption (small earthquakes and eruptions of dust and ash)
In 1997, Large eruptions continued with the dome collapsing and large pyroclastic flows affecting much of the island
Primary and Secondary effects of the Eruption
2/3 of the island was covered in ash50% of the population were evacuated to the north of the island to live in makeshift shelters 23 people died in 1997 Volcanic eruptions, pyroclastic flows and lahars have destroyed large areas of Montserrat. The capital, Plymouth, has been covered in layers of ash and mud. Floods as valleys were blocked with ash The airport and port were closed Farmland was destroyed and forest fires caused by pyroclastic flows Many schools and the only hospital was destroyed | As most of the southern area was destroyed any remaining inhabitants have had to endure harsh living conditions in the North.Transport remains a problem for people traveling to the island as the port and airport remain closed.The tourist industry is still suffering with few visitors except for cruise ships looking at the volcano Over half the population left the island and have not returnedMuch of the island is still uninhabitableBefore the eruption of 1995, over 12,000 people lived on the island but less than 5000 do today. |
Responses to the Eruption
Short-term responses.
- Evacuation of the southern part of the island
- Abandonment of the capital city.
- The British government gave £41 million in aid although riots occurred as locals complained that the British were not doing enough to help the island money for compensation and redevelopment.
- Unemployment rose due to the collapse of the tourist industry.
Long-term responses
- Money was given to individuals to help them move to other countries.
- An exclusion zone was set up in the volcanic region.
- New roads and a new airport were built.
- Services in the north of the island were expanded.
- The presence of the volcano resulted in a growth in tourism.
- The MVO (Montserrat Volcano Observatory) was set up to study the volcano and provide warnings for the future
- A Risk assessment was done to help islanders understand which areas are at risk and reduce problems for the future.
Still got a question? Leave a comment
Leave a comment, cancel reply.
Save my name, email, and website in this browser for the next time I comment.
Related links
- GCSE Geography Past Papers
Get a 9 in GCSE Physics with our Trusted 1-1 Tutors. Enquire now.
Let's get acquainted ? What is your name?
Nice to meet you, {{name}} what is your preferred e-mail address, nice to meet you, {{name}} what is your preferred phone number, what is your preferred phone number, just to check, what are you interested in, when should we call you.
It would be great to have a 15m chat to discuss a personalised plan and answer any questions
What time works best for you? (UK Time)
Pick a time-slot that works best for you ?
How many hours of 1-1 tutoring are you looking for?
My whatsapp number is..., for our safeguarding policy, please confirm....
Please provide the mobile number of a guardian/parent
Which online course are you interested in?
What is your query, you can apply for a bursary by clicking this link, sure, what is your query, thank you for your response. we will aim to get back to you within 12-24 hours., lock in a 2 hour 1-1 tutoring lesson now.
If you're ready and keen to get started click the button below to book your first 2 hour 1-1 tutoring lesson with us. Connect with a tutor from a university of your choice in minutes. (Use FAST5 to get 5% Off!)
Primary Hazards of Volcanoes
Volcanic eruptions produce a range of hazards - all of which can be devastating. The main primary hazards are:

- Ash fall (tephra) is a solid material of varying grain size (fine ash up to volcanic bombs) ejected into the atmosphere.
- Buildings often collapse under the weight of ash falling on to their roofs.
- Air becomes thick with ash and is very difficult to breathe in and can cause respiratory problems.
- The 2010 eruption of Mount Merapi in Indonesia is an example where volcanic ashfall affected the surrounding area.

Volcanic gases
- Volcanic gases can be poisonous.
- Examples include carbon dioxide, carbon monoxide, hydrogen sulphide, sulphur dioxide and chlorine.
- An example of an eruption that produced a lot of volcanic gases was the 1986 eruption of Lake Nyos, Cameroon.

Pyroclastic flows
- Pyroclastic flows are very hot (800 o C), have a high velocity (over 200km/h) and are a mixture of gases and tephra.
- Pyroclastic flows can destroy everything in their paths.
- The AD79 eruption of Mount Vesuvius in Pompeii, Italy.
- The 1980 eruption of Mount St. Helens in the USA.

- Lava flows are not usually a major threat when they are molten lava as they are slow moving.
- Lava flows create extensive areas of solidified lava.
- Southwest Iceland.
Secondary Hazards of Volcanoes
The main secondary hazards of volcanoes are:

- Lahars are fast mudflows that happen when rain mobilises deposits of volcanic ash.
- In 1985 the Armero tragedy took place when the Nevado del Ruiz volcano erupted in Colombia. Four lahars came towards the town of Armero and around 20,000 people died.

- A Jökulhlaup is the name of the flooding that happens when glaciers or ice caps melt. The term originates from Iceland where subglacial outburst floods happen at Vatnajökull.
1 Tectonic Processes & Hazards
1.1 Tectonic Processes & Hazards
1.1.1 Tectonic Plates
1.1.2 Distribution of Tectonic Hazards
1.1.3 Theoretical Frameworks
1.1.4 Earthquakes
1.1.5 Earthquake Hazards
1.1.6 Volcanoes
1.1.7 Tsunamis
1.1.8 End of Topic Test - Tectonic Processes
1.1.9 Exam-Style Question - Earthquakes
1.2 Natural Disasters
1.2.1 Introduction to Natural Disasters
1.2.2 Impacts of Hazards
1.2.3 Comparing Hazards
1.2.4 Development & Governance
1.3 Natural Disaster Case Studies
1.3.1 Tohoku Earthquake & Tsunami
1.3.2 Gorkha Earthquake
1.3.3 Mount Merapi Eruption
1.4 Trends & Patterns
1.4.1 Disaster Trends
1.4.2 Prediction
1.5 Disaster Modification
1.5.1 Hazard Management
1.5.2 Modifications
1.5.3 End of Topic Test - Natural Disasters
1.5.4 Exam-Style Question - Disaster Modification
2 Option 2A: Glaciated Landscapes & Change
2.1 Glaciated Landscapes Over Time
2.1.1 Timeline of Glacial Change
2.1.2 Natural Causes
2.1.3 Ice Distributions
2.2 Periglacial Landscapes
2.2.1 Periglacial Processes
2.2.2 Periglacial Landforms
2.3 Glacial Processes
2.3.1 Mass Balance
2.3.2 Glacial Movement
2.4 Glacial Landforms
2.4.1 Landscapes
2.4.2 Erosional Landforms
2.4.3 Erosional Landforms 2
2.4.4 Erosional Landforms 3
2.4.5 Depositional Landforms
2.4.6 Fluvioglaciation
2.5 The Future of Glaciated Landscapes
2.5.1 The Value of Glaciated Landscapes
2.5.2 The Value of Glaciated Landscapes 2
2.5.3 Threats to Glaciated Landscapes
2.5.4 Managing the Threats to Glaciated Landscapes
3 Option 2B: Coastal Landscapes & Change
3.1 Coastal Landscapes
3.1.1 The Wider Coastal Landscape
3.1.2 Geological Structure
3.1.3 Lithology & Vegetation
3.2 Coastal Erosion & Deposition
3.2.1 Shaping Coastlines
3.2.2 Coastal Formations
3.2.3 Sediment Transportation
3.2.4 Subaerial Processes
3.3 Coastal Risks
3.3.1 Sea Level Change
3.3.2 Coastal Retreat
3.3.3 Coastal Flooding
3.4 Managing Coastlines
3.4.1 Consequences of Coastal Recession
3.4.2 Engineering Management Approaches
3.4.3 Governance Approaches
4 Globalisation
4.1 Globalisation
4.1.1 Intro to Globalisation
4.1.2 Development of Globalisation
4.1.3 Economic Policy & Globalisation
4.1.4 Government Policy & Globalisation
4.1.5 International Organisations & Globalisation
4.1.6 Measuring Globalisation
4.1.7 TNCs & Globalisation
4.1.8 TNCs - Aramco & Anglo-Iranian Oil
4.2 Negatives of Globalisation
4.2.1 'Switched Off' Places
4.3 Global Shift
4.3.1 Global Shift for Developing Nations
4.3.2 Global Shift for Developing Nations 2
4.3.3 Global Shift for Developed Nations
4.3.4 End of Topic Test - Globalisation
4.4 Migration
4.4.1 Megacities
4.4.2 International Migration
4.4.3 Country Interdependence
4.5 Culture
4.5.1 Global Culture
4.5.2 Cultural Erosion
4.6 Measuring Development
4.6.1 Measuring Development
4.7 Responses to Globalisation
4.7.1 Tensions Caused by Globalisation
4.7.2 Government Control of Globalisation
4.7.3 Ethical Responses to Globalisation
4.7.4 End of Topic Test - Migration, Culture, & Response
5 Option 4A: Regenerating Places
5.1 Types of Economies
5.1.1 Economic Activity
5.1.2 Employment
5.1.3 Economic Activity - Health & Life
5.1.4 Economic Activity - Education & Inequality
5.2 Function of Places
5.2.1 Characteristics of Places
5.2.2 Changes in Places
5.2.3 International Influences on Places
5.2.4 Historic Influences on Places
5.2.5 The Perception of Places
5.2.6 Lived Experience & Attachment
5.3 Regeneration
5.3.1 Migration & Capital
5.3.2 Success & Regeneration
5.3.3 Tensions & Conflict in Communities
5.3.4 Regeneration in the UK
5.3.5 Local Government Policies
5.3.6 Regeneration Strategies
5.3.7 Measuring Regeneration Success
5.4 Regeneration Case Studies
5.4.1 Stratford
5.4.2 Salford Quays
5.4.3 Croyde
6 Option 4B: Diverse Places
6.1 Population Structure
6.1.1 The UK
6.1.2 Population Characteristics
6.1.3 Government & Change
6.2 Past & Present Connections
6.2.1 International Influence on Places
6.2.2 Historic Influences on Places
6.2.3 The Perception of Places
6.2.4 Image & Identity
6.3 Urban & Rural Spaces
6.3.1 Perceptions of Urban Places
6.3.2 Perceptions of Rural Places
6.3.3 Evaluating Living Space
6.4 Diversity
6.4.1 UK Migrations
6.4.2 Challenges
6.4.3 Tensions & Conflict
6.4.4 Wider Outcomes of Tensions
6.4.5 Managing Tensions
6.5 Urban & Rural Case Studies
6.5.1 Stakeholders
6.6 Case Study - Tower Hamlets
6.6.1 Background
6.6.2 Characteristics
6.6.3 Issues
6.7 Case Study - Sturton-le-Steeple
6.7.1 Background
6.7.2 Characteristics
6.7.3 Issues
7 The Water Cycle & Water Insecurity (A2 only)
7.1 Hydrological Processes Global to Local
7.1.1 Global Hydrological Cycle
7.1.2 Drainage Basin Hydrological Cycle
7.1.3 Water Budgets
7.1.4 River Regimes
7.1.5 Storm Hydrographs
7.2 Influences on the Water Cycle
7.2.1 Causes of Drought
7.2.2 Impacts of Drought
7.2.3 Causes of Flooding
7.2.4 Impacts of Flooding
7.2.5 Climate Change & The Water Cycle
7.2.6 Impacts of Climate Change
7.2.7 End of Topic Test - The Water Cycle
7.3 Water Insecurity
7.3.1 Water Stress
7.3.2 Causes of Water Insecurity
7.3.3 Water Insecurity Risk
7.3.4 Conflict Over Water
7.4 Water Supply Management
7.4.1 Hard Engineering Schemes
7.4.2 Sustainable Management
7.4.3 Water Treaties & Framework
7.4.4 End of Topic Test - Water Insecurity & Management
8 The Carbon Cycle & Energy Security (A2 only)
8.1 The Carbon Cycle
8.1.1 Carbon Stores
8.1.2 Carbon Stores 2
8.1.3 Biological Carbon
8.1.4 Atmospheric Carbon
8.1.5 Burning Fossil Fuels
8.2 Energy Consumption
8.2.1 Consumption
8.2.2 Access to Energy
8.2.3 Energy Players
8.2.4 Supply & Demand
8.2.5 Pathways
8.2.6 End of Topic Test - Carbon & Consumption
8.3 Alternative Energy
8.3.1 Unconventional Fuels
8.3.2 Non-Fossil Fuels
8.3.3 Non-Fossil Fuels 2
8.4 Growing Demand for Resources
8.4.1 Deforestation
8.4.2 Water
8.4.3 Climate Change
8.5 The Future
8.5.1 Future Uncertainty
8.5.2 Adaptation Strategies
8.5.3 Mitigation Strategies
9 Superpowers (A2 only)
9.1 Superpowers
9.1.1 Introduction to Superpowers
9.1.2 Superpowers - Economic Power & Size
9.1.3 Superpowers - Political & Military Power
9.1.4 Superpowers - Culture, Demography & Resources
9.1.5 Geo-Strategic Location
9.2 Hard & Soft Power
9.2.1 Hard & Soft Power
9.2.2 Emerging Powers - China Rivalry
9.2.3 Emerging Powers - Chinese Sources of Power
9.2.4 Emerging Powers - Brazil
9.2.5 Emerging Powers - Russia
9.2.6 Emerging Powers - India
9.2.7 Theories of Development
9.2.8 Power Case Studies: Chinese One Belt One Road
9.2.9 Power Case Studies: Pakistan Nuclear Arms
9.2.10 Power Case Studies: OPEC
9.3 IGOs, TNCs & Alliances
9.3.1 Superpowers & IGOs
9.3.2 Superpowers & TNCs
9.3.3 Superpowers & Global Action
9.3.4 Superpowers & Alliances
9.3.5 Superpowers & the Environment
9.4 Changing Global Influence
9.4.1 Recent Tensions between Powers
9.4.2 China in Africa & Asia
9.4.3 Tensions in the Middle East
9.4.4 Economic Problems for Superpowers
9.4.5 Future Pattern of Power
10 Option 8A: Health & Human Rights (A2 only)
10.1 Human Development
10.1.1 Measuring Development
10.1.2 Factors Improving Development
10.1.3 Variations in Health
10.2 Role of Governments & IGOs
10.2.1 Targets & Attitudes
10.2.2 Financial IGOs
10.2.3 The United Nations (UN)
10.2.4 The UN's MDGs & SDGs
10.3 Human Rights
10.3.1 Human Rights
10.3.2 Differences Between Countries
10.3.3 Transitions to Democracy
10.3.4 Differences Within Countries
10.3.5 The Demand for Equality
10.4 Interventions
10.4.1 Geopolitical Interventions
10.4.2 International Intervention Players
10.4.3 Evaluating Geopolitical Intervention
10.4.4 Military Interventions
10.4.5 Evaluating Military Interventions
10.5 Development Aid
10.5.1 Forms
10.5.2 Successes
10.5.3 Criticisms
10.5.4 Development Aid & the Environment
10.5.5 Evaluating Development Aid
10.5.6 Economic Inequalities
11 Option 8B: Migration & Identity (A2 only)
11.1 Globalisation & Migration
11.1.1 Intro to Migration
11.1.2 Trends in Migration
11.1.3 Common Migration Patterns
11.1.4 Causes of Migration
11.1.5 Restrictions on Migration
11.2 Consequences of Migration
11.2.1 Intro to Culture
11.2.2 Social & Demographic Tensions of Migration
11.2.3 Political & Economic Tensions of Migration
11.3 Nation States
11.3.1 Intro to Nation States
11.3.2 Borders
11.3.3 Nationalism
11.4 Responses to Global Migration
11.4.1 Responses to Global Migration
11.4.2 Global Organisations
11.4.3 IGOs and World Trade
11.4.4 Financial IGOs
11.4.5 Environmental IGOs
11.5 Sovereignty & Identity
11.5.1 Sovereignty & Nationalism
11.5.2 Complex Identities
11.5.3 Challenges to National Identities
11.5.4 Tensions within Nations
11.5.5 Failed States
Jump to other topics
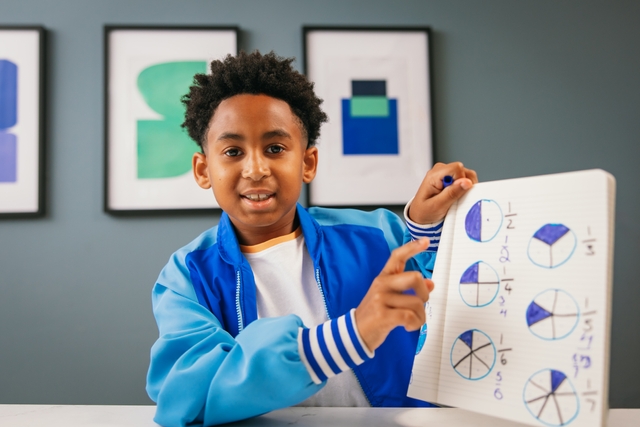
Unlock your full potential with GoStudent tutoring
Affordable 1:1 tutoring from the comfort of your home
Tutors are matched to your specific learning needs
30+ school subjects covered
Earthquake Hazards
Skip to content
Get Revising
Join get revising, already a member.
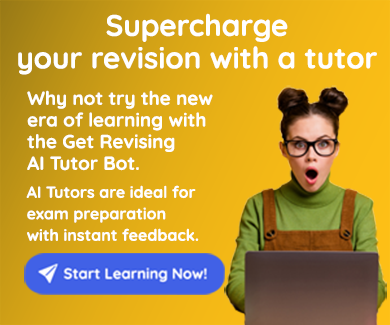
Volcanic Eruption CASE STUDIES
- Created by: Catherine Rice-Williams
- Created on: 22-04-13 13:11
- Plate tectonics
Report Thu 30th May, 2013 @ 16:02
Just what I was after, thanks!
Similar Geography resources:
Hazards case studies on spec 0.0 / 5
Hot spots 0.0 / 5
Earth Hazards 0.0 / 5
Monserrat volcanoes Case-Study 0.0 / 5
Hazards Case Studies - Yellowstone and Cameroon. 0.0 / 5
Volcano Case Study: Montserrat 5.0 / 5 based on 1 rating Teacher recommended
Plate Tectonics 1.0 / 5 based on 2 ratings
Montserrat 1995 Volcano Case Study 0.0 / 5
Geography A-Level - Physical - Volcanic Hazards 0.0 / 5
Mass Movement & Landslides 0.0 / 5

- TOP CATEGORIES
- AS and A Level
- University Degree
- International Baccalaureate
- Uncategorised
- 5 Star Essays
- Study Tools
- Study Guides
- Meet the Team
- Hazardous Environments
Chaiten Volcanic Eruption Case Study

Chaitén Eruption
Where did it happen? (written description, map of the location)
Chaitén is a small volcanic caldera (cauldron-like volcanic feature normally formed by the collapse of land following a volcanic eruption) located in southern Chile. The volcano is 3km in diameter and is located 17km west of the Michinmahuida volcano and 10km northeast of the town on Chaiten, hence the name of the volcano.
When did it happen? (date, duration, sequence of events, pace of management and recovery)
The largest and most recent eruption was in May 2008, but before this, the volcano consisted mainly of a rhyolitic lava dome which was last active 9400 years ago. On 2 nd May 2008, Chaitén began erupting violently, producing many plumes, pyroclastic flows and lahars, and creating a new lava dome on the north side of the old dome. Chaitén continued to erupt from 2 nd May to 8 th May with nearly continuous ash emission and irregular large explosions. The eruption has had serious consequences for the nearby town of Chaitén due to the effect s of lahars and ash, and the ash from the eruption even disrupted travel and agriculture in surrounding countries. The plume of steam and ash rose up to 16.8km into the atmosphere on the first day, and continued to do this on the 3 rd May. The plume on this day rose over the Andes Mountains, drifted over Argentina and spreads out across the Atlantic Ocean. Due to the ash, many schools and roads were closed- some of which were located hundreds of kilometres away from the volcano.

This is a preview of the whole essay
The Chilean Government Emergencies Office declared a red alert for Chaitén town at 2pm local time on 2 nd May. An evacuation of 1500 people from the town to shelters at Castro and Puerto Monnt began, with priority being given to the most vulnerable groups of people- the sick, the elderly and pregnant women. Further reports stated that the town’s supply of drinking water was suspended because of the danger of contamination from volcanic ash, so emergency water was brought in. The Army, Navy, Air Force, Police, Fire fighters and government were all involved in the emergency. On 3 rd May, it was reported that 1221 people were evacuated by the Chilean Navy, and a further 500 were to be evacuated by the end of the day. Further evacuations using ships with a capacity of 1000 people happened on the 4 th May. More water was brought in by the Navy on the 4 th May, and was given to the Futaleufu and Palena districts which were without portable water supplies.
During the first week of the eruption, 80% of Chaitén town was damaged- sediment from flood waters buried buildings up to 1.5m and there was damage to the airport and marine facilities. On 6 th May, another eruption happened which sent ash 30km high into the atmosphere. Due to this, the Chilean government ordered a total evacuation of people within 50km of Chaitén. The ash continued to affect air traffic in South America. The volcanic activity continued into February 2009, when a portion of the dome collapsed, sending a pyroclastic flow to within 4km of Chaitén town.
Why did it happen?
Chaitén is located above the Peru-Chile subduction zone, and in this convergent boundary, the Nazca plate is being subducted under the South American plate. There is more volcanic activity in the south of South America than the north, as the southern end of the plate is being subducted at a steeper angle than the north. The more dense Nazca plate is subducting under the western edge of the South American plate along the pacific coast of the continent, and this collision of plates is responsible for creating the Andes Mountains and the volcanoes. Because the oceanic lithosphere subducts beneath the continental lithosphere, a belt of volcanoes will be generated on continental crust, called a volcanic arc.
Who was affected by it happening?
The total population of Chaitén town was affected as everyone was evacuated away to other towns and cities. Due to the evacuation though, only 1 person died, and this was an elderly person who died at sea while en route for Puerto Montt. The environment and town was damaged more than the population. Forests near to the volcano were burned by pyroclastic flows and lateral explosions, and large parts of southern Argentina and Chile were coated with ash, which has had long term consequences for agriculture. This has not always been bad though, as the ash from the volcano adds new minerals to the soil. Lahars caused flooding in Chaitén town, depositing ash mud up to a metre in height. This damaged many buildings and filled the original course of the Chaitén River past the town. Because of this, the river created a new course through Chaitén town, which destroyed much of the town by July 2008.
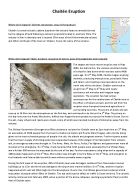
Document Details
- Author Type Student
- Word Count 818
- Page Count 2
- Level AS and A Level
- Subject Geography
Related Essays
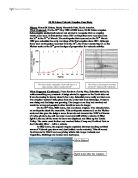
Mt St Helens Volcanic Eruption Case Study - Mount St Helens, Rocky Mountain...
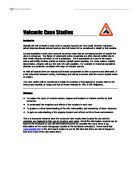
Volcanic Case Studies
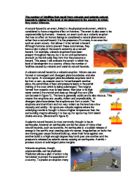

The number of fatalities that result from volcanic and seismic natural haza...
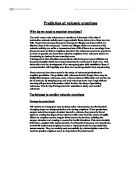
Prediction of volcanic eruptions
- International
- Education Jobs
- Schools directory
- Resources Education Jobs Schools directory News Search
A Level Geography Hazards Case Studies
Subject: Geography
Age range: 16+
Resource type: Other

Last updated
13 May 2021
- Share through email
- Share through twitter
- Share through linkedin
- Share through facebook
- Share through pinterest
This resource contains all the case studies required for the topic of Hazards for AQA A Level Geography.
Case studies included are:
- HIC Eyjafjallajokull volcanic eruption
- LIC Mount Merapi volcanic eruption
- HIC Kobe earthquake
- LIC Haiti earthquake
- HIC Japanese tsunami
- LIC Indonesian tsunami
- The Philippines (a multi-hazard environment)
- Australian wildfires
Each case study is organised into bullets points and therefore easy to read.
Tes paid licence How can I reuse this?
Get this resource as part of a bundle and save up to 27%
A bundle is a package of resources grouped together to teach a particular topic, or a series of lessons, in one place.
A Level Physical Geography Case Studies
This bundle includes all of the case studies for the topics of Water and Carbon Cycles, Coastal Systems and Landscapes and Hazards for **AQA** A Level Geography. Water and Carbon Cycle case studies: * The River Eden drainage basin * The Amazon rainforest Coastal Systems and Landscapes case studies: * The Holderness coastline * The Sundarbans Hazards case studies: * HIC Eyjafjallajokull volcanic eruption * LIC Mount Merapi volcanic eruption * HIC Kobe earthquake * LIC Haiti earthquake * HIC Japanese tsunami * LIC Indonesian tsunami * The Philippines (a multi-hazard environment) * Australian wildfires Each case study is organised into bullet points and is therefore easy to read.
Your rating is required to reflect your happiness.
It's good to leave some feedback.
Something went wrong, please try again later.
This resource hasn't been reviewed yet
To ensure quality for our reviews, only customers who have purchased this resource can review it
Report this resource to let us know if it violates our terms and conditions. Our customer service team will review your report and will be in touch.
Not quite what you were looking for? Search by keyword to find the right resource:
- 0 Shopping Cart
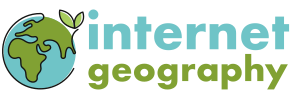
Soufrière Hills Volcano, Montserrat, West Indies.
Soufrière Hills Volcano , Montserrat, West Indies. Synopsis of events by former Montserrat resident, photographer and Author Lally Brown.
Where is Montserrat? Montserrat is a small tropical island of approximately 40 sq. miles in the Caribbean, fifteen minutes flying time from Antigua. It is a British Overseas Territory and relies on UK Government aid money to survive. It is of volcanic origin with the Soufrière Hills above the capital of Plymouth the highest point of the island.
How and when did the volcano erupt? Prior to 1995 the volcano in the Soufrière Hills had been dormant for 350 years but on the morning of 18th July 1995 steam and fine ash could be seen coming from the flanks of the Soufrière Hills accompanied by a roaring sound, described as being like a jet engine. In the capital of Plymouth there was a strong smell of ‘bad eggs’ the hydrogen sulphide being emitted by the awakening volcano.
Montserrat was totally unprepared. No-one had ever imagined the dormant volcano would erupt. The Soufrière Hills was the breadbasket of the island where farmers worked the fertile agricultural land, while the busy capital and island port of Plymouth nestled at the foot of the hills.
Scientists arrived from the University of the West Indies to assess the situation. They said the volcano was producing ‘acoustic energy explosions’ at approximately half-hour intervals sending ash and vapour three to four hundred metres into the air.
What happened next? Before July 1995 Montserrat was a thriving tourist destination with a population of 10,000 people but over several weeks there was a mass exodus from the island and a run on the banks with people withdrawing cash.
Several areas near the vent that had opened up in the hillside were declared exclusion zones and residents were evacuated to the safe north of the island into schools and churches.
It was evident the volcano was becoming more active when a series of small earthquakes shook the island. Heavy rain from passing hurricanes brought mudflows down the hillsides into Plymouth. Sulphide dioxide emissions increased, a sure sign of heightened activity.
The scientists hoped to be able to give a six hour warning of any eruptive activity but when they discovered the magma was less than 1 km below the dome they said this could not be guaranteed, saying there was a 50% chance of an imminent eruption. An emergency order was signed by the Governor and new exclusion zones were drawn with people evacuated north.
The years 1995 to 1997 The Soufrière Hills volcano became increasingly active and more dangerous.
Montserrat Volcano Observatory (MVO) was established to monitor activity and advise the Government.
December 1995 saw the first pyroclastic flow from the volcano.
The capital of Plymouth was evacuated for the last time in April 1996.
Acid rain damaged plants.
Two-thirds of Montserrat became the new exclusion zone , including the fertile agricultural land.
Population dropped to 4,000 with residents leaving for UK or other Caribbean islands.
Frequent heavy ashfalls covered the island with blankets of thick ash.
On the seismic drums at the MVO swarms of small hybrid earthquakes frequently registered. Also volcano-tectonic earthquakes (indicating fracture or slippage of rock) and ‘Broadband’ tremors (indicating movement of magma).
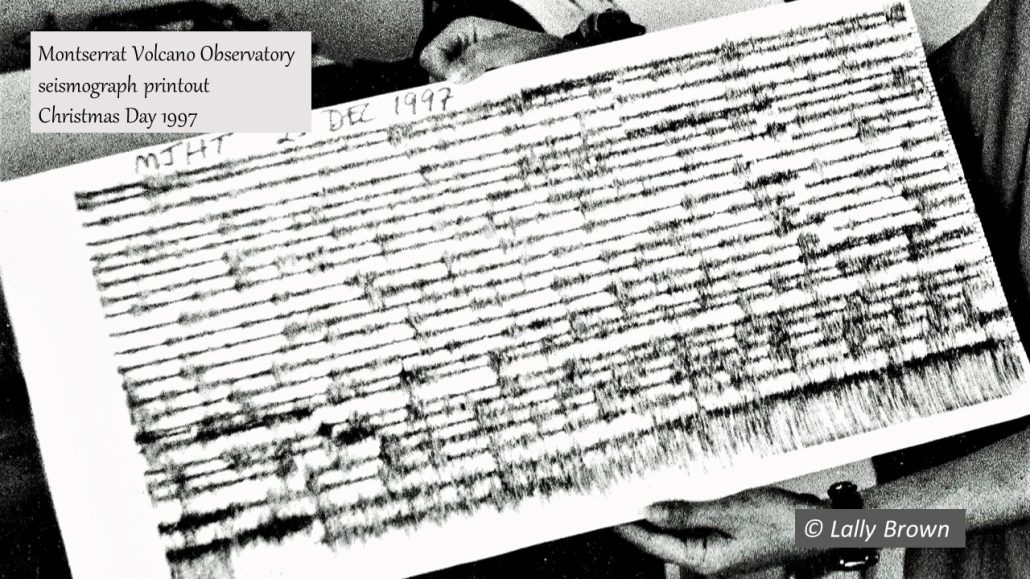
- Click to share
- Print Friendly
MVO Seismograph printout Dec 1997
‘Spines’ grew rapidly out of the lava dome to heights of up to 15 metres before collapsing back.
Rainfall caused dangerous mudflows down the flanks of the Soufrière Hills.
Temporary accommodation was built to house evacuees living in churches and schools.
25th June 1997 Black Wednesday For a period of twenty minutes at 12.59 pm the volcano erupted without warning with devastating consequences. A massive pyroclastic flow swept across the landscape and boulders up to 4 metres in diameter were thrown out of the volcano. Over 4 sq.km was destroyed including nine villages and two churches. The top 300ft had been blown off the lava dome. Tragically nineteen people were caught in the pyroclastic flow and died.
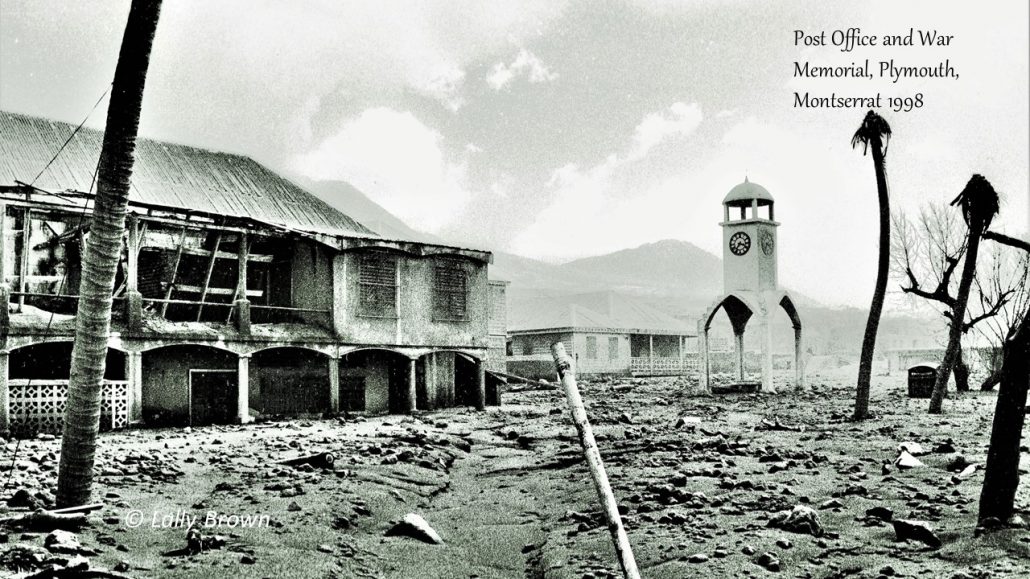
Post Office and War Memorial 1997
Lateral blast December 1997 Midnight on Christmas Day 1997 the MVO reported that hybrid earthquakes had merged into a near-continuous signal clipping the sides of the seismic drum. At 3am on Boxing Day there was a massive collapse of the dome. Approximately 55 million cubic metres of dome material shot down the flanks of the volcano into the sea. Travelling at speeds of 250-300 km per hour it took less than a minute to slice a 7 km wide arc of devastation across southern Montserrat. The evacuated villages of Patrick’s and O’Garros were blasted out of existence. A delta 2 km wide spilled into the sea causing a small tsunami .
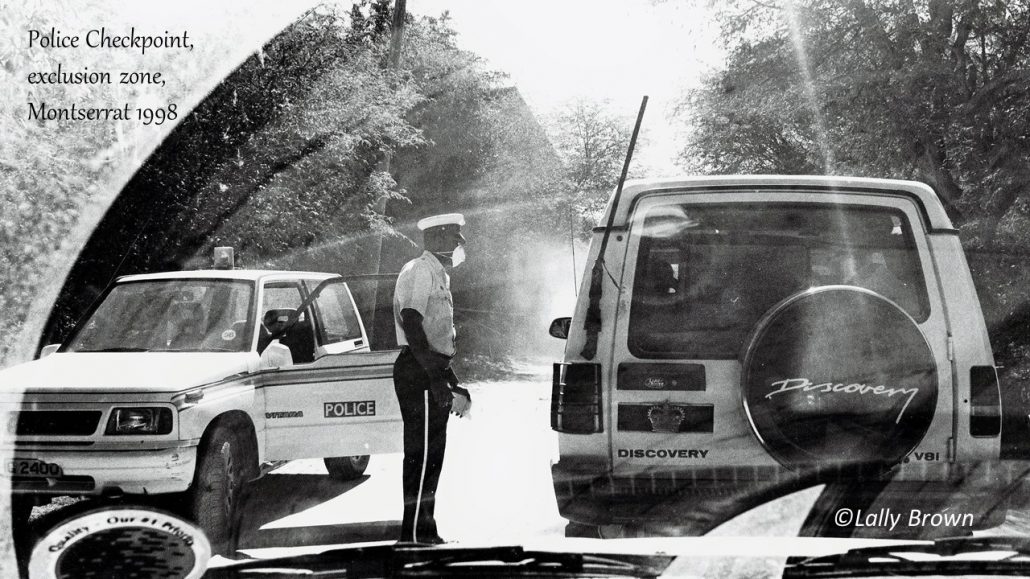
Police checkpoint Montserrat
March 1999 After a year of apparent inactivity at the volcano the Scientists declared the risk to populated areas had fallen to levels of other Caribbean islands with dormant volcanoes. Arrangements were made to encourage overseas residents to return. Plans were put in place to reopen the abandoned airport.
2000 to 2003 One year after the volcano had been declared dormant there was a massive collapse of the dome, blamed on heavy rainfall.
In July 2001 another massive collapse of the dome described as ‘a significant eruption’ caused airports on neighbouring Caribbean islands to close temporarily due to the heavy ashfall they experienced. A Maritime Exclusion Zone was introduced around Montserrat and access to Plymouth and the airport prohibited.
Soufrière Hills volcano was now described as a ‘persistently active volcano’ that could continue for 10, 20 or 30 years. (ie possibly to 2032).
In July 2003 ‘the worst eruption to date’ took place, starting at 8 pm 12th July and continuing without pause until 4 am morning of 13th July. Over 100 metres in height disappeared from the mountain overnight. It was the largest historical dome collapse since activity began in July 1995.
A period of relative quiet followed.
2006 The second largest dome collapse took place with an ash cloud reaching a record 55,000 metres into the air. Mudflows down the flanks of the Soufrière Hills was extensive and tsunamis were reported on the islands of Guadeloupe and Antigua.
Another period of relative quiet followed.
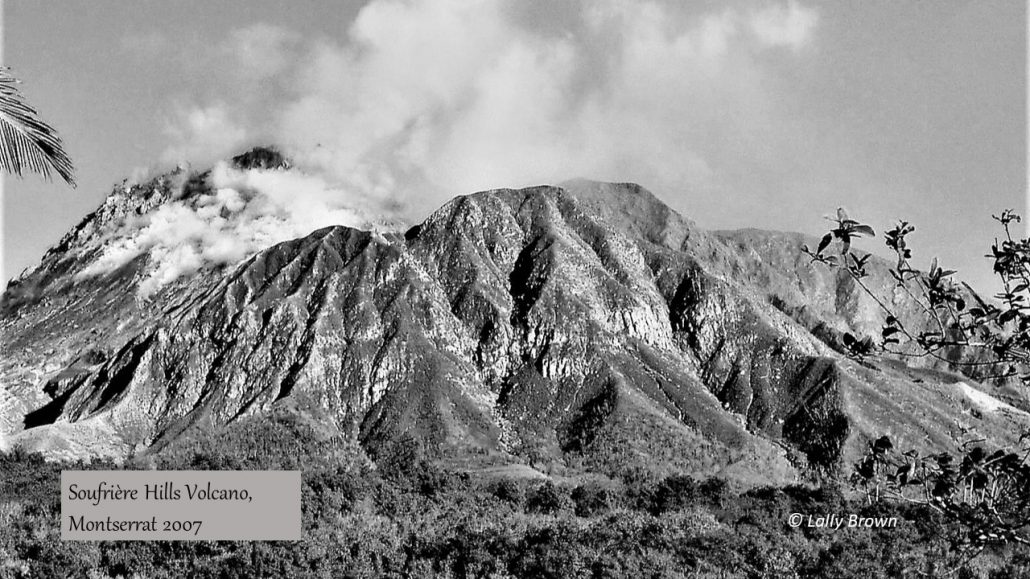
Soufriere Hills volcano 2007
2010 Another partial dome collapse with pyroclastic flows reaching 400 metres into the sea and burying the old abandoned airport. There was extensive ashfall on neighbouring islands.
Again followed by a period of relative quiet.
2018 Although the Soufrière Hills volcano is described as ‘active’ it is currently relatively quiet. It is closely monitored by a team at the Montserrat Volcano Observatory (MVO). They advise the Government and residents on the state of the volcano.
Negative effects of the volcano:
· Approximately two-thirds of Montserrat now inaccessible (exclusion zone);
· Capital of Plymouth including hospital, government buildings, businesses, schools etc. buried under ash;
· Fertile farming land in the south in exclusion zone and buried under ash;
· Population reduced from 10,000 to 4,000;
· Businesses left Montserrat;
· Tourism badly affected;
· Concern over long term health problems due to ash;
· Volcano Stress Syndrome diagnosed;
· Huge financial cost to British Tax Payer (£400 million in aid);
· Loss of houses, often not insured;
· Relocation to the north of Montserrat by residents from the south.
Positive effects:
· Tourists visiting Montserrat to see the volcano, MVO and Plymouth, now described as ‘Caribbean Pompeii’;
· Geothermal energy being investigated;
· Sand mining for export;
· Plans for a new town and port in north;
· New housing for displaced residents built;
· New airport built (but can only accommodate small planes);
· New Government Headquarters built;
· Businesses opening up in the north of the island;
· Ferry to Antigua operating.
Lally Brown
You can follow Lally Brown on Twitter.
If you are interested in reading a dramatic eyewitness account of life with this unpredictable and dangerous volcano then the book ‘THE VOLCANO , MONTSERRAT AND ME’ by Lally Brown is highly recommended. You can order a paper back or Kindle version on Amazon .
“As time moves on and memories fade, this unique, compelling book will serve as an important and accurate first-hand record of traumatic events, faithfully and sensitively recounted by Lally Brown.”
Prof. Willy Aspinall Cabot Professor in Natural Hazards and Risk Science, Bristol University.
Leave a Reply
Leave a reply cancel reply.
Your email address will not be published. Required fields are marked *
Save my name, email, and website in this browser for the next time I comment.
I agree to the terms and conditions laid out in the Privacy Policy
Notify me of follow-up comments by email.
Notify me of new posts by email.
This site uses Akismet to reduce spam. Learn how your comment data is processed .
Share this:
- Click to share on Twitter (Opens in new window)
- Click to share on Facebook (Opens in new window)
- Click to share on Pinterest (Opens in new window)
- Click to email a link to a friend (Opens in new window)
- Click to share on WhatsApp (Opens in new window)
- Click to print (Opens in new window)
Please Support Internet Geography
If you've found the resources on this site useful please consider making a secure donation via PayPal to support the development of the site. The site is self-funded and your support is really appreciated.
Search Internet Geography
Top posts & pages.

Latest Blog Entries

Pin It on Pinterest
Thank you for visiting nature.com. You are using a browser version with limited support for CSS. To obtain the best experience, we recommend you use a more up to date browser (or turn off compatibility mode in Internet Explorer). In the meantime, to ensure continued support, we are displaying the site without styles and JavaScript.
- View all journals
- Explore content
- About the journal
- Publish with us
- Sign up for alerts
- Open access
- Published: 16 July 2024
Lower ionospheric resonance caused by Pekeris wave induced by 2022 Tonga volcanic eruption
- Hiroyo Ohya 1 ,
- Fuminori Tsuchiya 2 ,
- Tamio Takamura 3 ,
- Hiroyuki Shinagawa 4 ,
- Yukihiro Takahashi 5 &
- Alfred B. Chen 6
Scientific Reports volume 14 , Article number: 15659 ( 2024 ) Cite this article
175 Accesses
Metrics details
- Environmental sciences
- Natural hazards
The submarine volcano Hunga Tonga–Hunga Ha’apai erupted explosively on January 15, 2022, offering a unique opportunity to investigate interactions between the atmosphere and ionosphere caused by Lamb and Pekeris waves. However, the resonance of Pekeris waves has not been previously detected. In this study, we applied a multi-point monitoring approach focusing on the lower ionosphere and atmospheric electric field. Here we show observed oscillations of 100–200 s in manmade transmitter signals and the magnetic and atmospheric electric fields, which were caused by Pekeris waves. However, no corresponding changes with the period of 100–200 s in atmospheric pressure due to Pekeris waves were observed on the ground. A simulation of neutral wind revealed Pekeris waves oscillating near the mesopause, suggesting resonance. Therefore, the oscillation in atmospheric electric field is interpreted that the resonance in the lower ionosphere was projected onto the Earth's surface via a global electric circuit.
Similar content being viewed by others
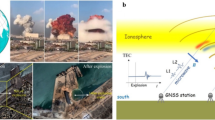
Atmospheric wave energy of the 2020 August 4 explosion in Beirut, Lebanon, from ionospheric disturbances

Resonant signals in the lithosphere–atmosphere–ionosphere coupling
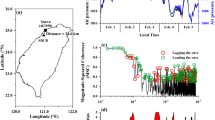
Large air pressure changes triggered by P-SV ground motion in a cave in northern Taiwan
Introduction.
Hunga Tonga–Hunga Ha’apai (HTHH), a submarine volcano in the southern Pacific Ocean (20.54 °S, 175.38 °W), erupted explosively during 04:00–04:15 UT on January 15, 2022 1 , 2 , with an estimated volcanic explosivity index of 5–6 (on a scale from 0 to 8), determined according to the ejecta volume, eruptive ash column height, and impulse seismic waves 3 . During this Plinian eruption, the volcanic plume reached a height of ~ 58 km 4 and the thermal energy released was estimated to range from ~ 1.0 × 10 19 to 2.8 × 10 19 J, comparable to the 1991 Pinatubo (~ 1 × 10 19 J) and 1883 Krakatoa eruptions (~ 3 × 10 19 J) 5 . Due to the large magnitude of the HTHH eruption, Lamb and Pekeris waves were generated 6 . Lamb waves are acoustic waves that propagate non-dispersively in the horizontal direction near the Earth’s surface, with a phase velocity of ~ 310 m/s 7 . Pekeris waves are resonance oscillations inherent to the Earth’s atmosphere, with a slower phase velocity of ~ 230–245 m/s 6 , 8 . Although Pekeris waves have been described as the L 1 mode of Lamb waves 9 , they have an anti-phase between the mesosphere and the stratosphere, whereas Lamb waves are vertically in-phase. The energy of Pekeris waves is enclosed between the stratopause and mesopause, within two atmospheric temperature minima, and the amplitudes of Pekeris waves are higher at the upper stratopause (> 45 km). Pekeris waves had never been observed within the 85 years since their theoretical prediction 10 until they were recorded by the Himawari-8 geostationary satellite following the HTHH eruption 6 .
The global electric circuit between the Earth’s surface and lower ionosphere 11 is driven by an electric power source consisting of lightning discharges, thunderclouds, precipitation, and electrified clouds. In fair weather, the air current flows downward from the ionosphere to the Earth’s surface, whereas it flows upward during thunderstorms. In studies of the global electric circuit, the atmospheric electric field, or potential gradient ( E z ), is typically observed using ground-based field mills. After the HTHH eruption, the variation in E z observed in the Czech Republic was similar to that in the amplitude of very low-frequency (VLF) transmitter signals, which are used to measure electron density changes in the D-region ionosphere (height, 60–90 km) 12 . Many studies have examined the F-region ionosphere in association with the HTHH eruption, but coupling between the D-region ionosphere and atmosphere due to Lamb and Pekeris waves generated by the HTHH eruption has not been demonstrated. In this study, we investigated variation in the D-region ionosphere using VLF/LF (3–30/30–300 kHz) transmitter signals, as well as Ez , ground-based magnetic field data, and ground-level atmospheric pressure data associated with the HTHH eruption (Fig. 1 ). In particular, the VLF/LF receiver at Tainan, Taiwan (TNN) belongs to the Asia VLF Observation (AVON) system in Southeast Asia 13 .

Map of the region influenced by the 2022 Hunga Tonga-Hunga Ha’apai (HTHH) eruption. ( A ) Very low-frequency/low-frequency (VLF/LF) transmitter signals, atmospheric electric field, ground-based magnetic field, and atmospheric pressure data. Brown star indicates the HTHH volcano; blue triangles indicate VLF/LF transmitters; red diamond indicates a receiver; green rectangle indicates location of atmospheric electric field and atmospheric pressure observations at CHB; purple circle indicates location of SORATENA atmospheric pressure observations at OKN; pink triangle indicates the ground-based magnetometer at KAK; red curves indicate distances from the HTHH volcano. ( B ) Enlarged map of an area near observation sites shown in ( A ).
Results and discussion
The amplitudes of three VLF/LF paths were processed using a high-pass filter (HPF), with a threshold > 1.67 mHz or < 10 min, and found to vary with the arrival times of both Lamb and Pekeris waves (Fig. 2 ). Based on the observed VLF/LF amplitudes, the estimated propagation velocities of the Lamb and Pekeris waves were ~ 307 and ~ 235 m/s, respectively, within the ranges of ~ 300–315 m/s and ~ 230–240 m/s reported in previous studies 6 , 11 , 12 , 13 . The amplitude of the VLF/LF variation due to Pekeris waves (2–4 dB) was higher than that due to Lamb waves (1–2 dB). Similarly, the amplitude of the magnetic field horizontal component ( B H ) (HPF: > 1.67 mHz) at Kakioka, Japan (KAK) was lower (± 0.8 nT) when Lamb waves arrived and higher (± 1.5 nT) when Pekeris waves arrived. However, atmospheric pressure (HPF: > 1.67 mHz, P ) observed by the SORATENA weather sensor at Okinawa (OKN) varied greatly around the Lamb wave arrival time (± 5 hPa), whereas amplitude variation was not observed at the Pekeris wave arrival time. The method for determining the arrival times of the Lamb (the blue vertical line) and Pekeris waves (the red vertical line) is as follows. First, we calculated the horizontal distance from the HTHH volcano to each observation site. For VLF/LF data, we adopted the shortest distance from the HTHH volcano to each propagation path. The distances for the B H at KAK, JJI-TNN, JJY60-TNN, BPC-TNN, and P at OKN were 7831.9 km, 8167.7 km, 8311.6 km, 8499.9 km, and 8032.3 km, respectively. Next, since the propagation velocity of Lamb waves estimated from VLF data was ~ 307 m/s, we calculated the propagation time of Lamb wave from distance and velocity. Therefore, the Lamb wave should arrive in the order of B H , P , JJI-TNN, JJY60-TNN, and BPC-TNN. The B H corresponds to the current in the lower ionosphere, the VLF/LF waves correspond to electron density fluctuations at a reflection altitude of approximately 90 km, and P is the atmospheric pressure at the surface of the Earth, so they are arranged in this order from top to bottom in Fig. 2 . The period of the VLF variation caused by Lamb waves was the same as that of atmospheric pressure (300–500 s), although the period of B H due to Lamb waves (500–1000 s) differed from that of VLF and atmospheric pressure. In contrast, the period of the VLF variation due to Pekeris waves (200–1000 s) for the three paths was similar to that of B H (300–1000 s). There was no clear signature in the P at the arrival time of the Pekeris wave (red vertical line). This is because the amplitude of Pekeris wave at the Earth’s surface is very small as suggested by theory 10 . At the D-region altitude (60–90 km), which is the reflection altitude of VLF/LF waves, the energy of Pekeris wave is trapped in the atmospheric temperature minimum region (50–110 km height), resonance occurs, and the amplitude of Pekeris wave increases. Therefore, no signatures of Pekeris waves can be seen in P at the Earth’s surface, although they can be seen in VLF/LF waves at the D-region altitude. These results demonstrate that Pekeris waves exhibited slight atmospheric pressure fluctuations at the Earth’s surface, although they were amplified at D-region altitudes.
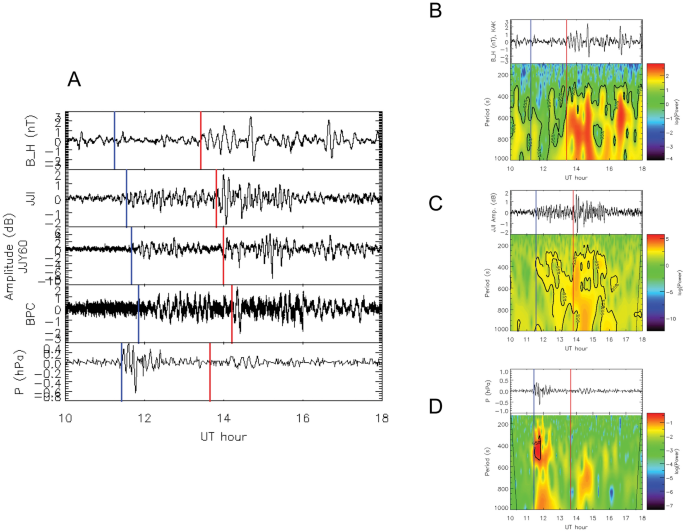
Signatures of Lamb and Pekeris waves in VLF/LF observations (high-pass filter [HPF]: > 1.67 mHz or < 10 min). ( A ) Horizontal (H) component of the magnetic field ( B H ) at KAK (top panel), VLF/LF amplitudes for the paths JJI–TNN (second panel), JJY60kHz–TNN (third panel), and BPC–TNN (fourth panel), and atmospheric pressure data at OKN (bottom panel). ( B ) Waveform of B H (top panel) and wavelet spectrum (bottom panel). ( C ) Waveform of JJI–TNN amplitude (top panel) and wavelet spectrum (bottom panel). ( D ) Waveform of atmospheric pressure at OKN (top panel) and wavelet spectrum (bottom panel). Blue and red lines indicate Lamb and Pekeris wave arrival times at each site, respectively.
Both E z (HPF: > 1.67 mHz or < 10 min) at Chiba, Japan (CHB), and B H at KAK also varied at the Pekeris wave arrival time, although the amplitudes of these fluctuations were low at the Lamb wave arrival time (Fig. 3 ). The amplitude of E z variation due to Pekeris waves (± 50 V/m) was higher than that due to Lamb waves (± 30 V/m). The periods of the variation in E z at CHB due to Lamb and Pekeris waves were 200–1000 and 100–1000 s, respectively, similar to the B H range (300–1000 s). In contrast, little variation in atmospheric pressure was observed at CHB due to Pekeris waves, despite large variation at the Lamb wave arrival time (± 0.6 hPa). The amplitudes of Pekeris waves are low on the ground but increase at altitudes above 30 km and become even larger at D-region altitudes 6 . The electron density in the lower ionosphere is influenced by Pekeris waves, leading to changes in the electric current that in turn alter B H . The air current within the global electric circuit is conveyed by atmospheric ions; therefore, variation in the current in the lower ionosphere is hypothesized to result in an increase or decrease in the number density of atmospheric ions, thereby causing shifts in the atmospheric electric field on the ground.
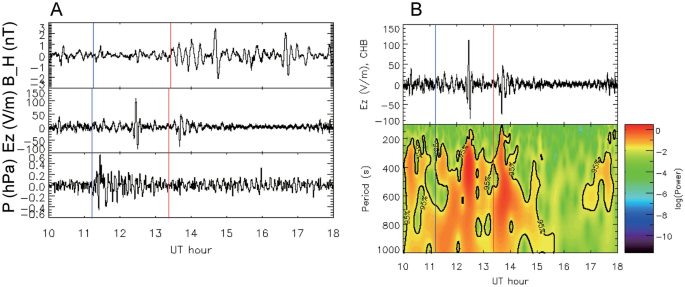
Signature of Pekeris waves in E z (HPF: > 1.67 mHz or < 10 min). ( A ) B H at KAK (top panel), E z at CHB (middle panel), and atmospheric pressure data at CHB (bottom panel). ( B ) Waveform of E z at CHB (top panel) and wavelet spectrum (bottom panel). Blue and red lines indicate Lamb and Pekeris wave arrival times at each site, respectively.
For Lamb waves, coherence values between VLF/LF amplitudes and other data ( B H and E z ) were < 0.4, although those for Pekeris waves were higher than those for Lamb waves (Fig. 4 ). The common periods among multiple VLF/LF paths were 146 s (frequency: 6.8 mHz) and 205 s (4.9 mHz) between the VLF/LF amplitudes and B H , and 171 s (5.8 mHz), 205 s, and 341 s (2.9 mHz) between the VLF/LF amplitudes and B H and E z . In the F-region ionosphere, atmospheric gravity waves (GWs) originating from the HTHH volcano and migrating by Lamb wave had an impact over a long period of time (~ several hours) and over a wide area, causing TID, etc. 15 , 16 , 17 , 18 , although duration of GWs migrated by Pekeris wave was short 14 . The Pekeris wave did not has strong effect for the F-region ionosphere. However, in the D-region ionosphere, which is the reflection height of VLF/LF waves, the Lamb and Pekeris waves, rather than GWs, directly changed the electron density. Pekeris wave had a relatively larger amplitude than Lamb wave at this altitude due to acoustic resonance in the neutral wind simulation (Fig. 5 ). Therefore, for Lamb wave, the B H and VLF/LF waves in the lower ionosphere were small and the period of fluctuation was slightly different (Fig. 2 ), while in the case of Pekeris wave, the B H and VLF/LF waves clearly fluctuated with the similar period. The E Z at the Earth’s surface was surprisingly similar to the B H variation for both Lamb and Pekeris waves. Although atmospheric ions near the Earth’s surface might change due to Lamb wave, it does not seem to result in large changes in the E z . It is possible that the lower ionosphere was greatly fluctuated by Pekeris wave with increased amplitude due to acoustic resonance, and the fluctuations in the lower ionosphere were projected to E z on the Earth’s surface via a global electric circuit. Therefore, these results demonstrate that Pekeris waves cause more D-region ionospheric variation than Lamb waves, and that the D-region ionosphere and E z on the ground varied with similar periods via the global electric circuit due to Pekeris waves.
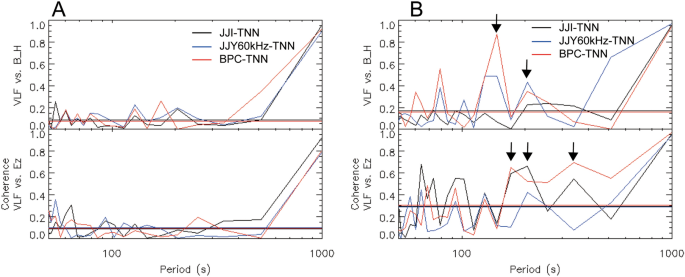
Coherences between VLF/LF amplitudes and other data (ground-based magnetic field at KAK and E z at CHB). ( A ) Coherence between VLF/LF amplitudes and B H at KAK (top panel) and coherence between the VLF/LF amplitudes and E z at CHB (bottom panel) for the Lamb wave. ( B ) Coherence between the VLF/LF amplitudes and B H at KAK (top panel) and coherence between the VLF/LF amplitudes and E z at CHB (bottom panel) for the Pekeris wave.
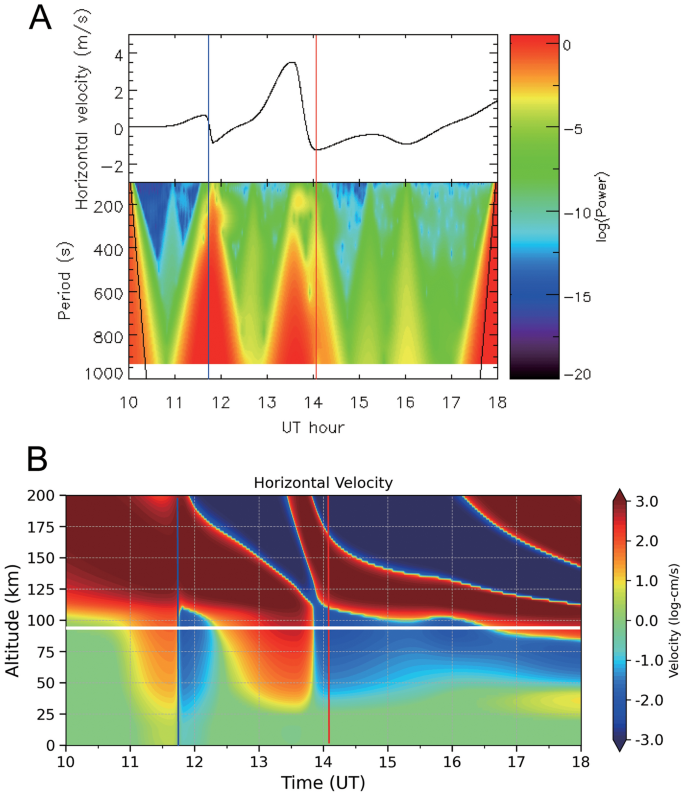
Simulation of horizontal velocity of neutral wind at the midpoint of the JJY60kHz–TNN path (28.3 °N, 125.2 °E) and the wavelet spectrum on January 15, 2022. ( A ) Horizontal velocity at a height of 90 km (top panel) and wavelet spectrum (bottom panel). ( B ) Horizontal velocity below a height of 200 km. Blue and red lines indicate Lamb and Pekeris wave arrival times estimated from observed VLF/LF variations, respectively. White horizontal line indicates an altitude of 90 km. To illustrate small velocities clearly, logarithms of the velocities in the unit of cm/s are taken.
In a neutral wind simulation 14 , Lamb and Pekeris waves were observed at 11:38 UT and 13:33 UT, respectively (Fig. 5 ). Figure 5 A shows that at an altitude of 90 km, the horizontal wind velocity of Pekeris waves is larger than that of Lamb waves. In Fig. 5 B, the red areas visible at 10:00–11:40 UT, 11:40–13:30 UT, 13:30–16:00 UT, and 16:00–18:00 UT above 100 km altitudes are GWs caused by the HTHH eruption. This shows that the GWs propagated within the thermosphere and reached the midpoint of the JJY60-TNN path. It looks like this because the first GW has a fast propagation speed of about 750 m/s in the thermosphere, and the subsequent GWs have a slower propagation speed. Below 100 km altitudes, Lamb wave arrived at 11:38 UT and Pekeris waves arrived at 13:33 UT. This can be seen by the sudden change from red to blue at an altitude of 100 km. The simulated arrival time of Lamb waves was in good agreement with the time estimated from VLF/LF observations, although the simulated arrival time of Pekeris waves was earlier than that of VLF waves. These results may have been influenced by background wind, as well as the fact that atmospheric temperature and density inhomogeneities along the VLF/LF propagation paths were not included in the simulation calculations. Lamb and Pekeris wave oscillations with periods of 100–200 s were observed in the horizontal velocity at a height of 90 km. These oscillations were caused by resonance between the stratopause (~ 50 km height) and lower thermosphere. The periods of variation in both the VLF/LF amplitudes and E z for Pekeris waves were similar to that of the resonance of 100–200 s shown in the simulation. In the simulation, the horizontal velocity of Pekeris waves (− 1.26 to 3.53 m/s) was higher than that of Lamb waves (− 0.87 to 0.86 m/s), which was in good agreement with the observed amplitudes of variation in VLF/LF waves and E z , where positive (negative) horizontal velocity values indicate outward (inward) direction relative to the HTHH volcano.
This study is the first to discuss the observation of Pekeris wave resonance in the lower ionosphere in VLF/LF waves, magnetic fields, and the atmospheric electric field. Simulations of neutral wind showed similar oscillations due to Pekeris waves, which were caused by resonance between the stratopause (~ 50 km height) and the lower thermosphere. The atmospheric electric field showed similar oscillations even in ground-based observations, because oscillations near the mesopause were projected onto the Earth’s surface via the global electric circuit.
VLF/LF transmitter signals
The VLF/LF transmitters used in this study were JJI (32.05 °N, 130.82 °E, the frequency: 22.2 kHz), JJY60kHz (33.47 °N, 130.18 °E, 60.0 kHz), Japan, and BPC (34.63 °N, 115.83 °E, 68.5 kHz), China. The receiver was one of AVON and located at Tainan (TNN), Taiwan (23.06 °N, 120.15 °E). The distance between the HTHH and the VLF/LF propagation paths was ~ 8000 – 10,000 km. The receiver detects amplitude and phase of manmade narrowband transmitter signals. These VLF/LF signals received by a monopole antenna are then amplified, filtered, and digitized by the receiving system. The VLF/LF data are 0.1-s sampling. The digital data is automatically transmitted to a processing facility of Tohoku University, Japan. Time synchronization is achieved by a locked-GPS oscillator. To remove noise, we took a 30-s moving average for the raw data.
Atmospheric electric field data
The atmospheric electric field has been observed with an EFM-100 field mill (Boltek) on the roof of a building on the campus of Chiba University (CHB, 35.63 °N, 140.10 °E), Japan, since 1 June, 2016. The dynamic voltage range is ± 20 kV/m with a 0.5-s sampling time. The cut-off frequency of the low-pass filter is 11 Hz. The distance from the HTHH was ~ 7790 km.
Atmospheric pressure data
We used two kinds of atmospheric pressure data. The atmospheric pressure data have been observed with a WS601-UMB Smart Weather Sensor (Lufft) at the same place of CHB with atmospheric electric field data. The data were 5-s sampling. The accuracy of the atmospheric pressure was ± 0.5 hPa.
Atmospheric pressure data at Okinawa (OKN, 27.34 °N, 128.57 °E), Japan collected by the SORATENA array were provided by Weathernews Inc. The data was 1-min. sampling. The distance from the HTHH was ~ 8032 km.
Coherence calculation
Coherence, coh ( ω ), is defined by the following formula:
where ω is the angular frequency; S xy ( ω ) is the cross-spectra of two discrete time signals; S xx ( ω ) and S yy ( ω ) are the spectra of x ( t ) and y ( t ), respectively; and K xy ( ω ) and Q xy ( ω ) are co- and quad-spectra, respectively 19 . Here, x ( t ) and y ( t ) denote the variation in the VLF/LF amplitudes for each path, and B H or E z , respectively.
K xy ( ω ) and Q xy ( ω ) are the real and imaginary parts of S xy ( ω ), respectively, and are calculated as follows:
Data availability
VLF/LF transmitter signal data were downloaded from https://pparc.gp.tohoku.ac.jp/research/vlf/ . Magnetic field data at Kakioka are available from https://www.kakioka-jma.go.jp/en/index.html . The SORATENA atmospheric pressure data in Japan are available upon request at https://global.weathernews.com/news/16551/ .
Shinbori, A. et al. Electromagnetic conjugacy of ionospheric disturbances after the 2022 Hunga Tonga-Hunga Ha’apai volcanic eruption as seen in GNSS-TEC and SuperDARN Hokkaido pair of radars observations. Earth Planets Space 74 , 106. https://doi.org/10.1186/s40623-022-01665-8 (2022).
Article ADS Google Scholar
Saito, S. Ionospheric disturbances observed over Japan following the eruption of Hunga Tonga-Hunga Ha’apai on 15 January 2022. Earth Planets Space 74 , 57. https://doi.org/10.1186/s40623-022-01619-0 (2022).
Poli, P. & Shapiro, N. M. Rapid characterization of large volcanic eruptions: Measuring the impulse of the Hunga Tonga Ha’apai explosion from teleseismic waves. Geophys. Res. Lett. 49 , e2022GL098123. https://doi.org/10.1029/2022GL098123 (2022).
Tonegawa, T. & Fukao, Y. Wave propagation of meteotsunamis and generation of free tsunamis in the sloping area of the Japan Trench for the 2022 Hunga-Tonga volcanic eruption. Earth Planets Space 74 , 159. https://doi.org/10.1186/s40623-022-01727-x (2022).
Wright, C. J. et al. Surface-to-space atmospheric waves from Hunga Tonga-Hunga Ha’apai eruption. Nature 609 , 741–746. https://doi.org/10.1038/s41586-022-05012-5 (2022).
Article ADS CAS PubMed PubMed Central Google Scholar
Watanabe, S., Hamilton, K., Sakazaki, T. & Nakano, M. First detection of the Pekeris internal global atmospheric resonance: Evidence from the 2022 Tonga eruption and from global reanalysis data. J. Atmos. Sci. 79 (11), 3027–3043. https://doi.org/10.1175/JAS-D-22-0078.1 (2022).
Matoza, R. S. et al. Atmospheric waves and global seismoacoustic observations of the January 2022 Hunga eruption, Tongo. Science 377 , 6601. https://doi.org/10.1126/science.abo7063 (2022).
Article CAS Google Scholar
Suzuki, T., Nakano, M., Watanabe, S., Tatebe, H. & Takano, Y. Mechanism of a meteorological tsunami reaching the Japanese coast caused by Lamb and Pekeris waves generated by the 2022 Tonga eruption. Ocean Model. 181 , 102153. https://doi.org/10.1016/j.ocemod.2022.102153 (2023).
Article Google Scholar
Francis, S. H. Global propagation of atmospheric gravity waves: A review. J. Atmos. Terr. Phys. 37 , 1011–1054. https://doi.org/10.1016/0021-9169(75)90012-4 (1975).
Pekeris, C. L. Atmospheric oscillations. Proc. R. Soc. Lond. 158A , 650–671. https://doi.org/10.1098/rspa.1937.0046 (1937).
Rycroft, M. J., Israelsson, S. & Price, C. The global atmospheric electric circuit, solar activity and climate change. J. Atmos. Solar-Terres. Phys. 62 , 1563–1576. https://doi.org/10.1016/S1364-6826(00)00112-7 (2000).
Article ADS CAS Google Scholar
Chum, J. et al. Atmospheric and ionospheric waves induced by the Hunga eruption on 15 January 2022: Doppler sounding and infrasound. Geophys. J. Int. 233 (2), 1429–1443. https://doi.org/10.1093/gji/ggac517 (2023).
Adachi, T. et al. Monitoring of lightning activity in Southeast Asia: Scientific objectives and strategies. Kyoto Work. Pap. Area Stud. 13 , 1–20 (2009).
Google Scholar
Shinagawa, H. & Miyoshi, Y. Simulation study of atmosphere–ionosphere variations driven by the eruption of Hunga Tonga-Hunga Ha’apai on 15 January 2022. Earth Planets Space 76 , 15. https://doi.org/10.1186/s40623-024-01960-6 (2024).
Wu, H., Lu, X., Wang, W. & Liu, H.-L. (2023) Simulation of the propagation and effects of gravity waves generated by Tonga volcano eruption in the thermosphere and ionosphere using nested-grid TIEGCM. J. Geophys. Res. Space Phys. 128 , e2023JA031354. https://doi.org/10.1029/2023JA031354 (2023).
Liu, L. et al. Concentric traveling ionospheric disturbances (CTIDs) triggered by the 2022 Tonga volcanic eruption. J. Geophys. Res. 128 , e2022J56. https://doi.org/10.1029/2022JA56 (2023).
Miyoshi, Y. & Shinagawa, H. Upward propagation of gravity waves and ionospheric perturbations triggered by the 2022 Hunga-Tonga volcanic eruption. Earth Planets Space 75 , 68. https://doi.org/10.1186/s40623-023-01827-2 (2023).
Vadas, S. L. et al. Traveling ionospheric disturbances induced by the secondary gravity waves from the Tonga eruption on 15 January 2022: Modeling with MESORAC-HIAMCMSAMI3 and comparison with GPS/TEC and ionosonde data. J. Geophys. Res. 128 , e2023JA031408. https://doi.org/10.1029/2023JA031408 (2023).
Hino, M. Spectral Analysis (Asakura Publishing, 1977) ( (in Japanese) ).
Download references
Acknowledgements
Atmospheric pressure data collected by the SORATENA array were provided by Weathernews Inc. Magnetic field data were obtained from the Kakioka Magnetic Observatory, Japan Meteorological Agency. Figures were prepared using Interactive Data Language (IDL) software provided by NV5 Geospatial Solutions.
This work was funded by Japan Society for the Promotion of Science (Grant no. 21K03679).
Author information
Authors and affiliations.
Graduate School of Engineering, Chiba University, Chiba, 263-8522, Japan
Hiroyo Ohya
Graduate School of Science, Planetary Plasma and Atmospheric Research Center, Tohoku University, Sendai, 980-8578, Japan
Fuminori Tsuchiya
Center for Environmental Remote Sensing, Chiba University, Chiba, 263-8522, Japan
Tamio Takamura
International Research Center for Space and Planetary Environmental Science, Kyushu University, Fukuoka, 819-0395, Japan
Hiroyuki Shinagawa
Graduate School of Science, Hokkaido University, Sapporo, 060-0808, Japan
Yukihiro Takahashi
Department of Physics, National Cheng Kung University, Tainan, 701, Taiwan
Alfred B. Chen
You can also search for this author in PubMed Google Scholar
Contributions
H.O. drafted the manuscript, analyzed VLF/LF waves, atmospheric electric field, ground-based magnetic data, and atmospheric pressure data, and calculated coherent coefficients. F.T., Y.T. and A.B.C. observed the VLF/LF waves. T.T. observed atmospheric pressure data. H.S. performed simulation of neutral winds. All authors contributed to the discussion of the results and the preparation of the manuscript.
Corresponding author
Correspondence to Hiroyo Ohya .
Ethics declarations
Competing interests.
The authors declare no competing interests.
Additional information
Publisher's note.
Springer Nature remains neutral with regard to jurisdictional claims in published maps and institutional affiliations.
Rights and permissions
Open Access This article is licensed under a Creative Commons Attribution 4.0 International License, which permits use, sharing, adaptation, distribution and reproduction in any medium or format, as long as you give appropriate credit to the original author(s) and the source, provide a link to the Creative Commons licence, and indicate if changes were made. The images or other third party material in this article are included in the article's Creative Commons licence, unless indicated otherwise in a credit line to the material. If material is not included in the article's Creative Commons licence and your intended use is not permitted by statutory regulation or exceeds the permitted use, you will need to obtain permission directly from the copyright holder. To view a copy of this licence, visit http://creativecommons.org/licenses/by/4.0/ .
Reprints and permissions
About this article
Cite this article.
Ohya, H., Tsuchiya, F., Takamura, T. et al. Lower ionospheric resonance caused by Pekeris wave induced by 2022 Tonga volcanic eruption. Sci Rep 14 , 15659 (2024). https://doi.org/10.1038/s41598-024-65929-x
Download citation
Received : 14 February 2024
Accepted : 25 June 2024
Published : 16 July 2024
DOI : https://doi.org/10.1038/s41598-024-65929-x
Share this article
Anyone you share the following link with will be able to read this content:
Sorry, a shareable link is not currently available for this article.
Provided by the Springer Nature SharedIt content-sharing initiative
By submitting a comment you agree to abide by our Terms and Community Guidelines . If you find something abusive or that does not comply with our terms or guidelines please flag it as inappropriate.
Quick links
- Explore articles by subject
- Guide to authors
- Editorial policies
Sign up for the Nature Briefing newsletter — what matters in science, free to your inbox daily.


IMAGES
VIDEO
COMMENTS
Twenty-seven of the 31 people injured in the eruption of the Whakaari/White Island volcano had burns to more than 30% of their body. The bodies of two victims have not been recovered and may have been lost to the sea. The ongoing seismic and volcanic activity in the area and subsequently heavy rainfall as well as low visibility and toxic gases ...
en the North American and Eurasian plate.Eyjafjallajökull is a 500m. long fissure volcano, that erupts. basalt. Basaltic lava is fluid in nat. r above the volcano.10km high a. h plume,containing sulphur, was emitted. This reached the stratosphere and caus. or air-travel in Europe.Some earthquakesFlooding from a jökulhau. river due to the me.
A Level Geography Eyjafjallajökull Case Study. 17 terms. Sophie2345678. Preview. Key Indicators of Development ... There were preparation plans in place for volcanic eruptions by the EU 500 farmers and their families were evacuated from around the Eyjafjallajökull area overnight authorities also evacuated an additional 700 people due to ...
Volcanoes case study 1 -Eyjafjallajökull. Tectonic setting of the hazard. The nature of the hazard (type, magnitude, frequency) Vulnerability. Capacity to cope (prediction, prevention, preparation) Institutional capacity. The impact of the event (social, economic, environmental), in the short and longer term.
1. Developing Country social impacts. 147 deaths and 120,000 made homeless, 15% of Goma destroyed by lava flows. Developing Country economic impacts. US$1.2 billion in economic losses and major international aid response launched. Study with Quizlet and memorize flashcards containing terms like Developed Country Eruption, Eyjafjallajokull year ...
Case Study - Mount Merapi, Indonesia. Name - Mount Merapi; Location - Java, Indonesia; Date - 25th October -30th November 2010; Magnitude - VEI 4 Plate boundary - Destructive plate boundary where the Indo-Australian plate is subducting below the Eurasian plate; Type of volcano - Stratovolcano or composite; Location of Mount Merapi. Impacts of the 2010 Eruption of Mount Merapi, Indonesia
Eyjafjallajokull is located below a glacier. The Eyjafjallajökull volcano erupted in 920, 1612 and again from 1821 to 1823 when it caused a glacial lake outburst flood (or jökulhlaup). It erupted three times in 2010—on 20 March, April-May, and June. The March event forced a brief evacuation of around 500 local people.
The volcano erupted at 8:32am on 18th May 1980. Effects - An earthquake caused the biggest landslide ever recorded and the sideways blast of pulverised rock, glacier ice and ash wiped out all living things up to 27km north of the volcano. Trees were uprooted and 57 people died.
This award-winning geography case study video resource reflects on the eruption of Eyjafjallajokull in 2010 and looks ahead to potential volcanic eruptions in Iceland. In this video, we cover: - The causes and impacts of the eruption, with visits to some of the localities directly affected. - Volcano monitoring and preparedness. - The impacts ...
The Secondary effects of the Eruption. 2/3 of the island was covered in ash50% of the population were evacuated to the north of the island to live in makeshift shelters 23 people died in 1997 Volcanic eruptions, pyroclastic flows and lahars have destroyed large areas of Montserrat. The capital, Plymouth, has been covered in layers of ash and mud.
The Eyjafjallajökull volcano erupted in 920, 1612 and again from 1821 to 1823 when it caused a glacial lake outburst flood (or jökulhlaup). It erupted three times in 2010—on 20 March, April-May, and June. The March event forced a brief evacuation of around 500 local people. Still, the 14 April eruption was ten to twenty times more ...
Ash fall (tephra) is a solid material of varying grain size (fine ash up to volcanic bombs) ejected into the atmosphere. Buildings often collapse under the weight of ash falling on to their roofs. Air becomes thick with ash and is very difficult to breathe in and can cause respiratory problems. The 2010 eruption of Mount Merapi in Indonesia is ...
The volcano had been dormant for over 300 years but started to erupt on the 18 th of July 1995. It started with warning signs of small earthquakes and eruptions of dust and ash. Following this Chances Peak remained active for five years. The most violent and intense eruptions occurred in 1997.
The eruption that began on 18 July 1995 was the first since the 19th century in Montserrat. When pyroclastic flows and mudflows began occurring regularly, the capital, Plymouth, was evacuated, and a few weeks later a pyroclastic flow covered the city in several metres of debris. Pyroclastic flows on the Soufriere hills were caused by the ...
Home > A Level and IB > Geography > Volcanic Eruption CASE STUDIES. Volcanic Eruption CASE STUDIES. 4.5 / 5 based on 5 ratings? Created by: Catherine Rice-Williams; Created on: 22-04-13 13:11; Fullscreen. Geography; Plate tectonics; ... OCR A Level Geography Geographical debates H481/03 - 17 Jun 2022 [Exam Chat] » ...
The largest and most recent eruption was in May 2008, but before this, the volcano consisted mainly of a rhyolitic lava dome which was last active 9400 years ago. On 2nd May 2008, Chaitén began erupting violently, producing many plumes, pyroclastic flows and lahars, and creating a new lava dome on the north side of the old dome.
Volcano eruption case study 1. Where and When? A volcanic eruption of Mount Ontake took place on September 27, 2014. Mount Ontake is a volcano located on the Japanese island of Honshu around 100 kilometres northeast of Nagoya and around 200 km southwest of Tokyo. Volcano type: Stratovolcano
Between 25th-26th October 2010, Mt Merapi erupted three times; thousands were evacuated from a 20km radius around the slopes of the volcano. The column of smoke rose vertically to 1.5km and pyroclastic activity began to subside, 18 people were found dead. The deaths were due to burns and respiratory problems. Between 17th-29th October 2010, the ...
1997. which year was considered the real 'disaster' year? chances peak. the volcanic area in Montserrat, where this volcano was is...? small tremors. why was no one too concerned at the beginning? phreatic eruption. what type of eruption was this? - (ash and steam forced out of small holes and cracks in the volcano) montserrat volcano observatory.
Nyiragongo Case Study. This case study has been developed to support students studying Edexcel B GCSE Geography. Tectonic Setting and Location. Mount Nyiragongo is a composite volcano located in the east of the Democratic Republic of the Congo (DRC). The volcano consists of a huge (2km wide) crater, usually filled with a lava lake, and is only 20km away from the city of Goma.
this creates many subduction zones and active volcanoes - approximately 25 active volcanoes with frequent eruptions and earthquakes. -It lies in a belt of tropical cyclones, and experiences a tropical monsoon climate and is therefore subject to heavy rainfall. Steep slopes combined with heavy rainfall mean that mass movements of earth are common.
Age range: 16+. Resource type: Other. File previews. pdf, 75.7 KB. This resource contains all the case studies required for the topic of Hazards for AQA A Level Geography. Case studies included are: HIC Eyjafjallajokull volcanic eruption. LIC Mount Merapi volcanic eruption.
Soufrière Hills volcano was now described as a 'persistently active volcano' that could continue for 10, 20 or 30 years. (ie possibly to 2032). In July 2003 'the worst eruption to date' took place, starting at 8 pm 12th July and continuing without pause until 4 am morning of 13th July.
During this Plinian eruption, the volcanic plume reached a height of ~ 58 km 4 and the thermal energy released was estimated to range from ~ 1.0 × 10 19 to 2.8 × 10 19 J, comparable to the 1991 ...